Penn Engineering’s Aleksandra Vojvodic is Catalyzing Ideas for Clean Energy
For nearly half a century, chemists, physicists and engineers have dreamed of a “hydrogen economy.” In that hypothetical world, the universe’s most plentiful atom would be the cornerstone of energy generation and storage; no more digging complex hydrocarbons out of the ground, only to burn them in processes that foul the planet’s air and water. Hydrogen, transported across the gradient of a fuel cell, could power cars, ships and cities, and do so cleanly and in perpetuity.
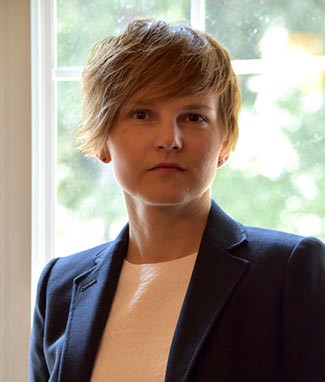
The key to this dream is efficiently splitting hydrogen off from the common molecules in which it’s found; if that process takes more energy than the hydrogen can later produce, nothing is gained. As plentiful as the atom is — two in every molecule of water — there is a formidable energy barrier in the way of isolating it.
One of Penn Engineering’s newest faculty members, Aleksandra Vojvodic, the Skirkanich Assistant Professor of Innovation in the Department of Chemical and Biomolecular Engineering, aims to crash through that barrier.
“You can do almost anything on this planet if you have enough energy,” Vojvodic says, “so we’re trying to find materials that lower the amount of energy you need.”
Using chemical intuition and solving the complex quantum mechanical equations of a material at the atomic level, Vojvodic employs supercomputers to churn through hundreds and thousands of candidate materials, some of which do not yet exist. The goal: to rationalize and design chemical reactions that not only liberate hydrogen from water, but capture the leftover oxygen as well.
Vojvodic’s area of expertise is materials design, in particular in the field of catalysis. Catalysts are materials that increase the rate at which chemical reactions can occur and lower the amount of energy required for the chemical transformation to take place. A key aspect of the catalyst material is that it participates in the chemical reaction without being consumed itself. A catalyst changes the way the reactant molecules interact with each other and the chemical routes that the intermediate chemical fragments take to form the desired product.
Decades of research produced a gamut of specialized catalysts, and they are ubiquitous in industrial processes, like the production of ammonia that is a precursor for fertilizers, and in everyday technology, like the exhaust systems of cars. However, materials that can efficiently split water, remain elusive. New search techniques are necessary.
“Materials science has existed for thousands of years, even if it wasn’t called that,” Vojvodic said. “Smiths and other artisans were working with metals and trying to understand their properties, though it was essentially through trial and error. Now we have more control. Once we can rationalize why a material works in a particular environment, we can start to fine tune it.”
Trained in physics in Sweden’s Lund University and Chalmers University of Technology, Vojvodic takes a theoretical approach to this problem. Understanding the thermodynamic and kinetic properties of a given arrangement of atoms, and armed with increasingly powerful computation tools to model them, she and her colleagues can predict the behavior of materials that have yet to be synthesized, or those made for completely unrelated purposes and never tested as catalysts.
Her work as staff scientist at the SLAC National Accelerator Laboratory and postdoctoral work at the Chemical Engineering Department at Stanford University in the SUNCAT Center for Interface Science and Catalysis earned her a spot in MIT Technology Review’s 2016 “35 Innovators Under 35.”
She and her colleagues at SUNCAT recently published research on a new catalyst that operates on the oxygen side of the water-splitting reaction and can survive harsh chemical environments. Their paper, which appeared in the journal Science, details an iridium-based catalyst that performs an oxygen evolution reaction with record-breaking efficiency in the acidic conditions. Such a catalyst could have a direct impact on improving water electrolyzers that use polymer electrolyte membranes, a recently developed technology found in fuel cells that overcomes many of the disadvantages of conventional alkaline electrolyzers.
“When developing new catalyst materials,” Vojvodic says, “we not only want them to be efficient, but for practical purposes we also need them to be cheap. In other words, we would like to avoid scarce elements as ruthenium and iridium. So my work has also involved looking for efficient oxygen evolution catalysts that use only earth-abundant elements.”
Earlier this year, Vojvodic led a computational team that collaborated with experimentalists from University of Toronto to develop such a catalyst. That study was also published in Science.
Now at Penn, Vojvodic has set her sights on finding not only new catalyst materials but also layered and 2D nano-structured materials for electronic, technological and environmental applications that will help change the energy landscape.
Her catalyst research will continue aiming not only to find new catalysts but also combinations of catalysts that can work on both sides of the hydrogen/oxygen equation at the same time. Having control of the oxygen chemistry in a water-splitting reaction could be useful in a variety of other industrial chemical processes, as well as conversion of nitrogen and other abundant molecules, and fuel cells, but the process is more complicated than simply mixing together two catalysts that work separately.
“Sometimes unexpected things happen when we mix two materials,” Vojvodic says. “The final product doesn’t always behave as something in between. The important thing to keep in mind for catalysis is that all the reactions are happening on the surface — you need to understand what the nanostructure of the catalyst looks like and how molecules are actually arranged at the surface of the material to understand what’s actually driving the reaction which is due the interaction between the surface and the reactant. The chemistry and the geometry have to combine in the right way to find a balance between stability and activity.”
Coming to a highly collaborative, interdisciplinary environment like Penn was important to Vojvodic — bringing new perspectives together in close proximity is itself a catalyst for innovation.
“Sometimes we theorists don’t know where to start,” Vojvodic says, “so we’ll do a big computational screening study that builds up databases of a few thousand materials. Hopefully you come with one, two or five candidates for the particular application we have in mind, then you knock on the door of your best friend experimentalist and work together to figure out if any of those compounds are stable. If that is the case, you can start investigating its chemical and physical properties.”
Collaboration has more fundamental benefits, too.
“There’s a synergistic effect,” Vojvodic says. “Unless someone challenges you to explain your thinking, you might not challenge yourself.”
Originally published at www.seas.upenn.edu.