Penn Engineers’ Magnetic Micro-robots Use Capillary Forces to Coax Particles into Position
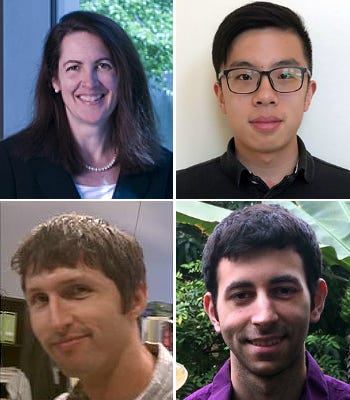
At microscopic scales, picking, placing, collecting and arranging objects is a persistent challenge. Advances in nanotechnology mean that there are ever more complex things we’d like to build at those sizes, but tools for moving their component parts are lacking.
Now, new research from the University of Pennsylvania’s School of Engineering and Applied Science shows how simple, microscopic robots, remotely driven by magnetic fields, can use capillary forces to manipulate objects floating at an oil-water interface.
This system was demonstrated in a study published in the journal Applied Physics Letters.
The study was led by Kathleen Stebe, Richer & Elizabeth Goodwin Professor in Penn Engineering’s Department of Chemical and Biomolecular Engineering, and Tianyi Yao, a graduate student in her lab. Nicholas Chisholm, a postdoctoral researcher in Stebe’s lab, and Edward Steager, a research scientist in Penn Engineering’s GRASP lab contributed to the research.
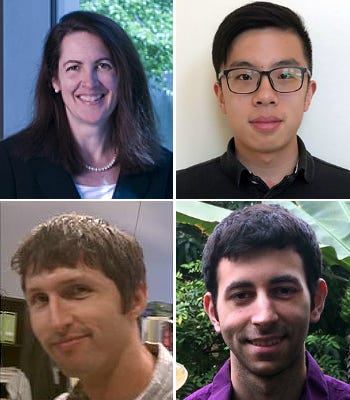
The micro-robots in the Penn team’s study are thin slices of magnet, about a third of a millimeter in diameter. Despite having no moving parts or sensors of their own, the researchers refer to them as robots because of their ability to pick and place arbitrary objects that are even smaller than they are.
That ability is a function of the specialized environment where these micro-robots work: at the interface between two liquids. In this study, the interface is between water and hexadecane, a common oil. Once there, the robots deform the shape of that interface, essentially surrounding themselves with an invisible “force field” of capillary interactions.
The same capillary forces that draw water from the roots of a tree to its leaves are here used to draw plastic microparticles into contact with the robot, or other particles already stuck to its edges.
“We’ve used these capillary forces to assemble things before, but now the robots and the particles are much lighter and a few orders of magnitude smaller in diameter,” Stebe says. “When you move down to the micron scale, it means that a different kind of physics governs the distortions. Collecting and organizing objects that are a few tens of microns across is quite an achievement, and not something that we’re going to be able to do by hand.”
The study demonstrated the physics governing the interactions between these micro-robots and the plastic particles they were tasked with manipulating.
“In the past,” Stebe says, “we took static objects and made distortions around them, then showed how particles were attracted to ‘high curvature’ regions of those distortions. Now, instead of a static object, we have a magnet that serves as a mobile distortion source.”
“This makes things more complicated,” Chisholm says. “As the robot moves toward particles, it creates a flow field that pushes the particles away, so now there’s hydrodynamic repulsion and capillary attraction interacting. The particles follow the energy minimum, which might mean moving uphill.”
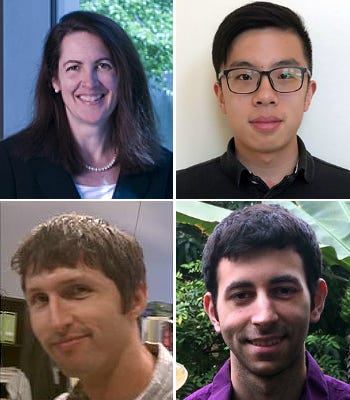
With a square-shaped robot, the researchers saw that once they got particles over the crest of the deformation, they were strongly attracted to the corners. This is a potentially useful property, as the robots could approach their targets from a wide range of angles and orientations and still end up with the particle in a predictable location.
“We’ve shown that when you change the robot shape, you change the type and strength of the interactions,” Stebe says. “Sharp corners hold onto the particles like grim death, but when we soften the corners, we can just give the robots a spin to release them.”
In addition to a soft-cornered square, the researchers also experimented with a round robot, as well as a flower-shaped one. All had the added advantage of being able to precisely release their cargo by spinning in place, with the flower-shaped robot’s “petals” providing the most precise control over the location of a cargo particle.
Finally, the team demonstrated a docking station. Consisting of a static piece of wavy plastic, the docking station is part above and part below the interface. This arrangement provides a very predictable set of distortions where the material crosses the interface.
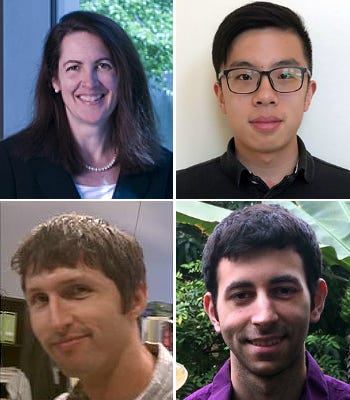
“We can move these robots around and collect things,” Steager says, “building up really complicated materials by picking up the pieces one at a time and docking them where we want.”
Because the interactions between the robots and particles have nothing to do with the materials they’re made out of, a wide array of applications are possible.
“The particles we’re manipulating in this study are about the average size of a human cell or smaller,” Yao says, “so this kind of system might have applications in the field of single-cell biology, with a magnetic micro-robot moving individual cells through different stages of an experiment.”
“These particles could also be part of a sensor system,” he says. “If you had a robot and sensor particles on an interface, you could collect those particles and carry the whole assembly toward the targeting area with an extremely fine degree of spatial control. In this scenario, a very low concentration of sensor particles is needed and they can be easily retracted after the test.”
Future work will involve developing a larger library of micro-robot shapes and behaviors for manipulating objects in their environment, as well as more robust sensing and control systems that would allow a greater degree of autonomy for the robots.
The research was supported by the National Science Foundation through grant DMR-1607878.