Aleksandra Vojvodic: Leveraging Chemistry for a Green World
By Lida Tunesi
Many of the technologies that could facilitate a sustainable future already exist. The idea for a hydrogen fuel cell, a technology that produces electricity without carbon emissions, has been around for over 100 years. The cost and lifetime of fuel cells, however, have prevented them from becoming ubiquitous. To complicate things further, fuel cells are only as green as the production of their hydrogen fuel. That means that renewable hydrogen sources such as water-splitting need to become economical enough to replace production from natural gas.
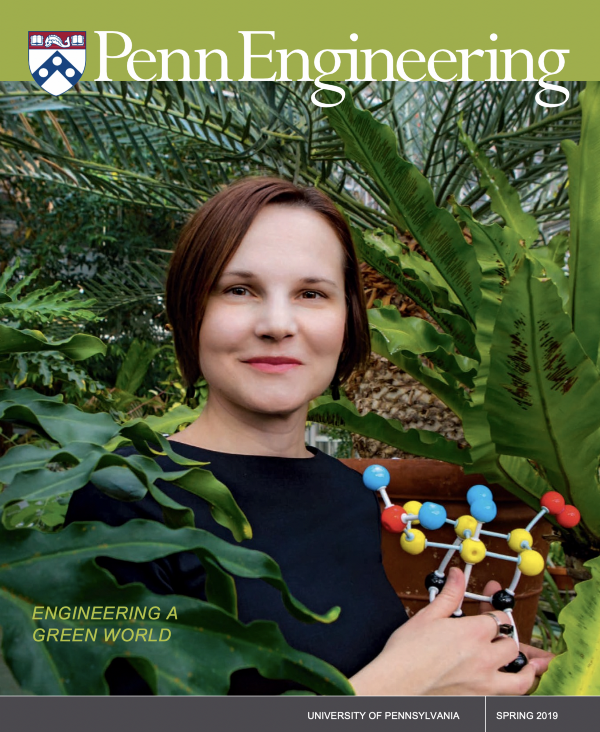
Fortunately, these challenges all have something in common. At their core they are chemistry problems, and each can be tackled by taking things to the atomic level. “All these systems — electrocatalysis, batteries, fuel cells — they all have something happening at the atomic scale. Protons, electrons and ions are moving around. It’s not only energy being transferred; there’s a process going on that is chemical in nature,” says Aleksandra Vojvodic, Skirkanich Assistant Professor of Innovation in Chemical and Biomolecular Engineering.
Vojvodic uses quantum mechanics to model the chemical reactions happening in these technologies as opposed to performing the reactions in a laboratory. By solving equations that describe chemical systems, Vojvodic is able to work on crucial energy and environmental issues in a completely virtual way.
STORING ENERGY
In theory, reusable batteries can store and dispense energy again and again, saving the energy and materials it would take to make more. But in reality, batteries degrade over time. Vojvodic is collabo- rating on two different projects to address a myste- rious cause of this degradation. The collaborations were formed via the Scialog program, which brings together multidisciplinary teams of researchers who have never worked together before to undertake scientific challenges.
With one group, Vojvodic is studying batteries with liquid electrolytes, such as the lithium-ion battery you might find in your cell phone or an electric vehicle. The other team is focused on solid-state batteries: heavier, stationary energy storage. Both projects are focusing on the electrolyte-cathode interface, where a layer of an unidentified material forms over time.
“Nobody knows what it is,” Vojvodic says, “and it’s very hard to see what’s happening there. Our experimental collaborators can see only the before and after. But we can model it. We can run and solve these quantum mechanical equations that let us study the interaction molecule by molecule.”
With the combined effort of her lab’s theory work and her experimental collaborators, Vojvodic is working out the chemical mechanism that forms this layer and is developing ways to stabilize the problem. The work on solid-state batteries is familiar territory, Vojvodic says, as she studied solid-state physics as an undergraduate. Her later work as a staff scientist at the SLAC National Accelerator Laboratory at Stanford University developed her expertise in solid-liquid interfaces.
Despite her physics background, Vojvodic was never tempted to go into fields like astronomy or high-energy physics. “I wanted to be more rooted on this planet. Being aware of climate issues has always been one of my interests.”
CATALYZING SUSTAINABILITY
In combating climate issues, Vojvodic is up against long-standing infrastructure such as gasoline- powered engines and utilities that run on natural gas. Hydrogen fuel provides an alternative, but hopes for a “hydrogen economy” largely hinge on developing efficient and sustainable sources of hydrogen.
One method is to split water into its hydrogen and oxygen components using electricity; a “green” process if the electricity comes from a renewable source. Catalysts can make this process more efficient by lowering the amount of energy the reactions require and speeding up the reactions. Catalysts are not consumed in a reaction and can be used again and again. Vojvodic and her students are developing catalysts for both the hydrogen- and oxygen-forming parts of the reaction. An efficient water-splitting method could also benefit the production of ammonia, a compound made of nitrogen and hydrogen, as this process currently sources most of its hydrogen from natural gas.
Ammonia production also accounts for about 2 percent of the world’s energy use — in other words, just over the same amount of energy as all buses, trains, and two- and three-passenger vehicles worldwide combined. It is a key ingredient in the fertilizers that help our crops grow and is a precursor to many industrial chemicals, so while we might not stop making it, Vojvodic thinks we can find a better method.
The process uses so much energy partly because it requires high temperatures and pressure. Many plants, however, are pros at fixating nitrogen under tamer conditions, using enzymes in their roots. One of Vojvodic’s projects seeks a way to imitate plants’ methods in order to make ammonia at a lower energy cost.
Ideally, all of these endeavors could come together in a chain of environmentally friendly links. “If we can get electricity by collecting sunlight, and use that electricity to split water and make hydrogen, then we can combine that hydrogen with nitrogen to make ammonia fertilizers to grow our crops,” Vojvodic says.
But she realizes that solutions are never as simple as they seem. Platinum, for instance, is a common catalyst in fuel cells but is a relatively rare element. Cobalt is more abundant but its mining is laden with issues of health, safety and child labor. “You can solve one problem, but in reality, things are more complex than that,” Vojvodic says. “There are always multiple factors. How earth-abundant is your material, how cheap, how efficient, and what is its lifetime? All of these have to come into your equation, and there are trade-offs.”
MODELING SOLUTIONS
How, then, to solve such a complicated equation of needs? Armed with the tools of her physics background and the power of supercomputers, Vojvodic uses quantum mechanics to model and test thousands of materials faster than she could in a chemistry lab. This lets Vojvodic do “virtual experiments” on materials’ viability as catalysts, and build up fundamental knowledge of structure- reactivity relationships.
“We do a lot of calculations, then collect the data and look for patterns,” Vojvodic says. “If you have a few thousand of these computational observations, you can start to make some conclusions about what makes a material work.”
Vojvodic has developed a protocol that helps new lab members get started in the computational aspects of the work. For the classroom, she developed a new course, Computational Science of Energy and Chemical Transformations, to teach graduate and advanced undergraduate students the same skills. The curriculum teaches concepts behind atomic-scale modeling and allows students to try it hands-on, using an educational allotment of Vojvodic’s NSF supercomputer time. The class culminates in a final project in which students work on a real-world problem in catalysis or energy.
Vojvodic looks forward to expanding her work to encompass even more of these fundamental issues. One area of interest is green chemistry, a field devoted to finding new chemical methods that use and produce less hazardous material and result in less waste, both in labs and on an industrial scale. Vojvodic is also interested in clean-water chemistry: finding ways to filter heavy metals and organic compounds from water sources.
“That’s what drew me to computational, atomic-scale work,” Vojvodic says. “There are many problems that you can work on, because everything is about the chemistry and physics of the materials.”