Testing is key to understanding and controlling the spread of COVID-19, which has already taken more than four million lives around the world. However, current tests are limited by the tradeoff between accuracy and the time it takes to analyze a sample.
Another challenge of current COVID-19 tests is cost. Most tests are expensive to produce and require trained personnel to administer and analyze them. Testing in low-and middle-income communities has therefore been largely inaccessible, leaving individuals at greater risk of viral spread.
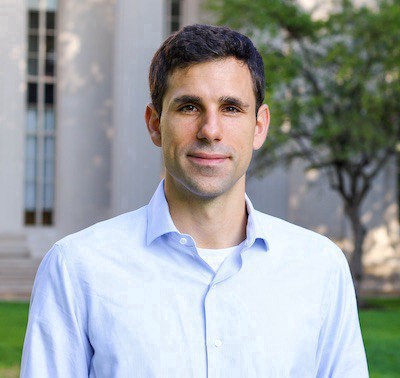
To address cost, time and accuracy, a new electrochemical test prototype, developed by Penn researchers in the School of Engineering and Applied Science and the Perelman School of Medicine, uses electrodes made from graphite — the same material found in pencil lead.
Led by César de la Fuente, Presidential Assistant Professor in Bioengineering, Microbiology and Psychiatry with a secondary appointment in Chemical and Biomolecular Engineering, their research has produced electrodes that reduce the cost to $1.50 per test and require only 6.5 minutes to deliver 100-pecent-accurate results from saliva samples and up to 88 percent accuracy in nasal samples.
While his previous research highlights the invention of RAPID (Real-time Accurate Portable Impedimetric Detection prototype 1.0), a COVID-19 testing kit which uses screen-printed electrodes, this new research published in PNAS presents LEAD (Low-cost Electrochemical Advanced Diagnostic), using the same concept as RAPID but with less expensive materials. De la Fuente’s current test reduces costs from $4.67 per test (RAPID) to $1.50 per test (LEAD) just by changing the building material of the electrodes.
“Both RAPID and LEAD work on the same principle of electrochemistry,” says de la Fuente. “However, LEAD is easier to assemble, it can be used by anyone and the materials are cheaper and more accessible than those of RAPID. This is important because we are using an abundant material, graphite, the same graphite used in pencils, to build the electrode to make testing more accessible to lower-income communities.”
This figure, adapted from the paper, shows the functionalization steps of LEAD which prepares the electrodes to bind to the sample. The height of the peaks indicates whether the sample is negative or positive. Because the SARS-CoV-2 spike protein in a positive sample binds to the electrode, it inhibits the emitted signal and produces a smaller peak.
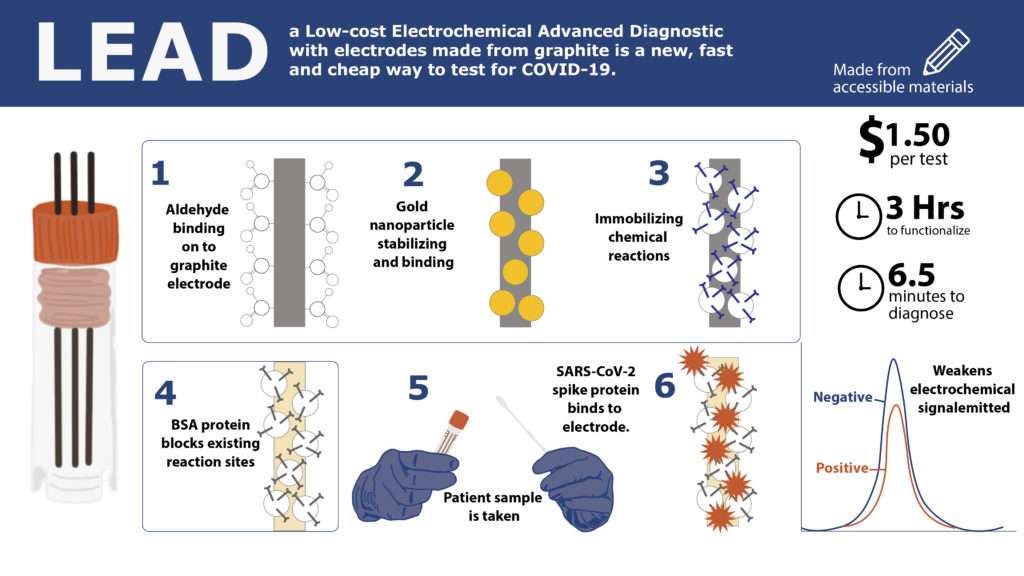
LEAD’s functionalization and sample diagnosis take less than a few hours and can be made for a fraction of some of the most inexpensive tests on the market. Future steps for LEAD are twofold.
“Currently, we are working to improve the technology and stability of our tests,” says de la Fuente. “We will always be looking for ways to make the most effective version of LEAD, but we are also working to find industry partners and conduct more clinical studies to push the use of LEAD for COVID testing as soon as possible.”
While COVID-19 is the top priority, de la Fuente’s tests can also detect other transmissible diseases, keeping this research relevant in the future.
“Another area of future research for our test design is multiplexing, which will allow us to detect multiple viruses and bacteria in a sample,” says de la Fuente. “Once COVID is relatively controlled, we can use LEAD to detect the flu, Herpes, bacterial infections, and even certain biomarkers.”
LEAD’s cost and time efficiency may help it become one of, if not the first, electrochemical COVID-19 tests on the market in the near future and its fundamental process of sample detection could keep it on the market indefinitely.
This research was funded by the National Institutes of Health under award number R35GM138201, the Nemirovsky Prize, and funds provided by the Dean’s Innovation Fund from the Perelman School of Medicine at the University of Pennsylvania.