The Perfect Storm
Lee Bassett’s Quantum Research is Making Waves
By Jacob Williamson-Rea
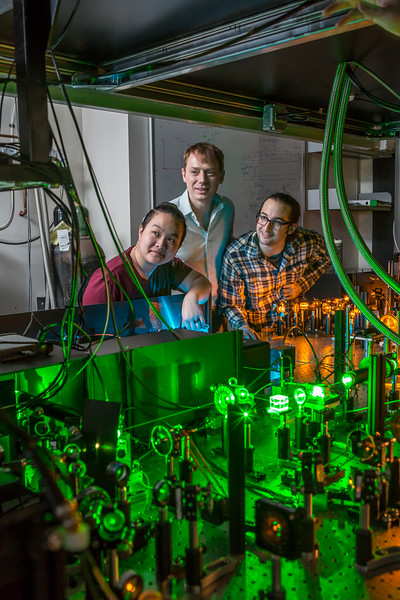
Lee Bassett can usually be found in his Quantum Engineering Laboratory at Penn Engineering, or enjoying time with his family and occasionally playing the tuba or piano in local music groups. But when a perfect storm produces large waves at the Jersey Shore, Bassett and lab member David Hopper head to the coast to surf.
“Waves carry energy, but we don’t talk about physics while we’re in the water,” Hopper says. “We shut it off and have fun, but the connection is there. Quantum physics is basically wave mechanics with complex numbers.”
The laws of quantum physics govern the submicroscopic world within individual atoms; revolutionary new kinds of computing, sensing and communication are being built around the laws’ unusual properties. For example, the quantum-mechanical model postulates that electron behavior can be mathematically understood by treating electrons as waves of matter, rather than particles. Quantum technologies need to measure and manipulate the quantum states of these waves, but the physical conditions required are extremely difficult to produce, requiring a protected-yet-accessible microscopic environment in which individual quantum states can be initialized, made to interact, and measured. Most quantum systems rely on ultra-cold temperatures and high vacuums to remain stable, limiting them to highly controlled laboratory settings.
Bassett’s team recently discovered a new quantum system in a two-dimensional material, which functions at room temperature and can be coupled to other devices. While exploring defects in hexagonal boron nitride (h-BN), a one-atom thick semiconductor material, the researchers observed an optical effect where individual electrons respond to an applied magnetic field. That effect depends on the electrons’ spin, which is a quantum- mechanical property that can be used to define a quantum bit, or qubit, the building block of quantum technologies.
“The balance between isolating and controlling a quantum system is a constant trade-off,” says Bassett, assistant professor in the Department of Electrical and Systems Engineering. “Quantum systems need to preserve quantum properties for a significant amount of time, which means they need to be isolated. At the same time, we need to control and interact with the system. That’s the challenge, and spin states have proven to be a good solution.”
Because h-BN doesn’t require extreme conditions or expensive equipment to keep its qubits stable, this discovery opens up an array of possible quantum technologies.
Bassett led the study along with Annemarie Exarhos, who was a post-doc in his lab and who now holds a faculty position at Lafayette College. Lab members David Hopper and Raj Patel also contributed to the study, as did Marcus Doherty of The Australian National University. Their results have been published in Nature Communications.
Quantum World Computing
Quantum computers promise to tackle complex problems at lightning speeds, enabling applications like rapidly simulating the physical world at the molecular level. Their promise lies in the fundamentally different way in which they represent information. Classical computers use transistors to store data and perform calculations with binary bits, electronic signals that represent 0 or 1. Quantum computing will instead rely on qubits, which use subatomic states such as the spin of an electron, to represent a 0, 1 or a superposition of both at the same time.
Classic binary bits are like a coin on a flat surface, always either heads or tails. Qubits, on the other hand, are like a flipping coin suspended in mid-air, allowing them to represent 0 and 1 simultaneously until they are measured. Along with entanglement, or the ability of physically separated states to influence one another, these properties allow quantum systems to store more information and process certain calculations much faster than classical computers.
But because measuring a qubit causes its 0-and-1 superposition to collapse, quantum systems are extremely fragile. Certain arrangements of atoms, like the defects Bassett and his colleagues discovered in h-BN, can serve as insulated, protected pockets for qubits. And because h-BN is two-dimensional, the qubits they contain are easily accessible.
2D Key
Quantum labs, including Bassett’s, have been exploring other materials with room-temperature qubits based on defects. Bassett’s lab also studies defects in the crystalline lattice of diamonds, which are known to harbor spin states that act as excellent quantum memories, quantum communication links and nanoscale quantum sensors.
Diamond-based technology is poised to be useful for quantum systems that need to withstand extreme conditions. But bulk materials pose other challenges; in particular, it is difficult to create and locate precise arrangements of defect qubits on the atomic scale within a 3D crystal.
The discovery of analogous defect-bound qubits in a 2D material, such as h-BN, can address this challenge. Because h-BN is only one atom thick, the qubits are always on its surface, and tools are available to directly image and control individual atoms. Not only does this make the spins accessible to researchers, it also makes the spins accessible to other quantum states in nanoscale devices, which would help create applicable quantum technology.
Bassett and his team will take this research even further thanks to the JEOL NEOARM transmission electron microscope housed at Penn’s Singh Center for Nanotechnology. The NEOARM, the only micro- scope of its kind in the country, can visually image single atoms, which will allow the researchers to develop a more complete understanding of h-BN defects and even create new ones.
“There are long-term, exciting goals involving this system,” Basset says. “This could eventually enable all sorts of technology, because the material offers unprecedented levels of control.”
Quantum Quakers
Hopper, who will be the first student to graduate with a Ph.D. from Bassett’s Quantum Engineering Lab, says that while he’s grateful for his advisor’s surfing knowledge, he is most thankful for the environment he creates in his lab.
“Lee does a really great job of bringing together people who enjoy working with others,” Hopper says. “He encourages us to leverage one another’s strengths, which makes it really easy to build and share expertise. He’s always encouraging discussions and collaborations, which makes for such a healthy environment, not only for the lab to grow, but for individuals to grow as well.
“Whether it’s in the lab or in the water, Lee’s one of those people who is so knowledgeable about anything that interests him.”
Bassett says that he too is grateful for the students in his lab, attributing their success to the overall environment at Penn, as well as the lab’s diverse background.
“There’s such an intellectually rich atmosphere at Penn,” Bassett says. “Our lab is a diverse mix of people who come from backgrounds in materials science, quantum physics, photonics and device engineering, but also from biomedicine, chemistry and more. This is the perfect storm for pioneering quantum research, because quantum science and technology encompasses so many disciplines, all while sort of defying a clear definition. Penn’s overall environment aligns with that idea, which helps to make our lab unique and successful.”