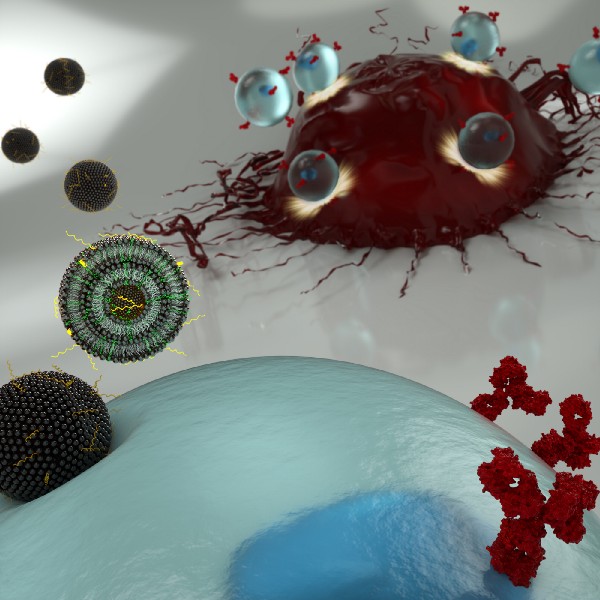
New cancer immunotherapies involve extracting a patient’s T cells and genetically engineering them so they will recognize and attack tumors. This technique is a true medical breakthrough, with an increasing number of leukemia and lymphoma patients experiencing complete remissions since CAR T therapy was FDA approved in 2017.
This type of therapy is not without challenges, however. Engineering a patient’s T cells is laborious and expensive. And when successful, the alterations to the immune system immediately make patients very sick for a short period of time, with symptoms including fever, nausea and neurological effects.
Now, University of Pennsylvania researchers have demonstrated a new engineering technique that, because it is less toxic to the T cells, could enable a different mechanism for altering the way they recognize cancer.
Treatment courses with T cells that use this mechanism could have fewer side effects for patients.
The researchers’ new engineering technique involves ferrying messenger RNA (mRNA) across the T cell’s membrane via a lipid-based nanoparticle, rather than using a modified HIV virus to rewrite the cell’s DNA. Using the former approach would be preferable, as it only confers a temporary change to the patient’s immune system, but the current standard method for getting mRNA past the cell membrane can be too toxic to use on the limited number of T cells that can be extracted from a patient.
The researchers demonstrated their technique in a study published in the journal Nano Letters. It was led by Michael Mitchell, Skirkanich Assistant Professor of Innovation in the Department of Bioengineering in Penn’s School of Engineering and Applied Science, and Margaret Billingsley, a graduate student in his lab.
They collaborated with one of the pioneers of CAR T therapy: Carl June, the Richard W. Vague Professor in Immunotherapy and director of the Center for Cellular Immunotherapies in the Abramson Cancer Center and the director of the Parker Institute for Cancer Immunotherapy at Penn’s Perelman School of Medicine
CAR T therapy involves engineering a patient’s own T cells so they express chimeric antigen receptors, the “CAR” of “CAR T,” on their surfaces. These receptors allow the T cells to recognize cancerous cells as foreign invaders and clear them from the body. Current engineering techniques involve removing a number of a patient’s T cells, rewriting their DNA with a virus so they express these CARs, then injecting them back into the patient.
“This viral engineering method produces T cells with permanent CAR expression, but that leads to severe adverse effects, as the CAR T cells remain active in the patient even after eradicating cancer cells,” says Billingsley. “Using mRNA to generate CAR T cells, however, creates T cells with transient CAR expression. This could allow clinicians to administer CAR T cell therapies in doses to target cancer cells without damaging as many healthy cells in the process, thus mitigating the side effects.”
Such an approach has not yet gained clinical traction, as methods for getting mRNA into T cells are still limited. The current standard, electroporation, which involves perforating the cell membrane with an electric pulse, is not an attractive option, as the highly invasive process has a high risk of killing the T cell or impacting its functionality. Even when optimized, electroporation often results in cell death in 50 percent of the cells during the cell manufacturing process.
Given the cost, difficulty and stakes associated with acquiring these cells from a CAR T patient, a much less toxic method of introducing mRNA is needed before this technique is a viable alternative to the DNA-editing approach.
Mitchell, Billingsley and their colleagues thus set out to find a suitable delivery platform for sneaking mRNA past the T cell’s membrane in sufficient quantities for them to be translated into the desired receptor proteins.
However, as T cells do not readily take up material from their environment, finding the right nanoparticle for this application was a challenge. Further, it would be crucial that the platform include both highly efficient delivery and low cytotoxicity — a rare combination.
“When delivering therapeutic mRNA into cells, you always need to balance potency with toxic side effects,” Mitchell says. “Our lab engineers ionizable lipid nanoparticles that can safely cross cellular membranes, but release therapeutic mRNA specifically when it needs to be released. We see this as a huge benefit, as the current clinical standard, electroporation, kills a large portion of the patient’s T cells.”
“Furthermore,” he says, “we envision as a platform technology for T cell delivery, as we can make mRNAs for different therapeutic CARs, or other therapeutic receptors, very quickly by simply altering the mRNA sequence, and therefore having a variety of therapeutic applications.”
With in vitro experiments, the researchers showed that their nanoparticles generated CAR T cells that were as effective at killing cancer cells as the virally engineered CAR T cells currently in clinical use. Next steps will include in vivo studies on the impacts of this delivery system, examining the effects of transient CAR expression on therapeutic efficacy and side effects.
“Given the increasing toolbox of RNA therapeutics, nanoparticle delivery of RNA has broad applications for T cell engineering including genetic editing of T cell DNA and modulation of protein expression,” says June.
Nathan Singh, a postdoctoral researcher, and Pranali Ravikumar, a research specialist, both of the June lab, and Rui Zhang, a postdoctoral researcher in the Mitchell lab, also contributed to the study.
It was supported by a Burroughs Wellcome Fund Career Award at the Scientific Interface, a U.S. National Institutes of Health Director’s New Innovator Award (DP2 TR002776), a grant from the American Cancer Society (129784-IRG-16–188–38-IRG), an Abramson Cancer Center-School of Engineering and Applied Science Discovery Grant (P30 CA016520), a 2018 Abramson Cancer Center-Bayer Innovation and Discovery Grant (18–80–44-MITC), and a Tau Beta Pi Graduate Research Fellowship.