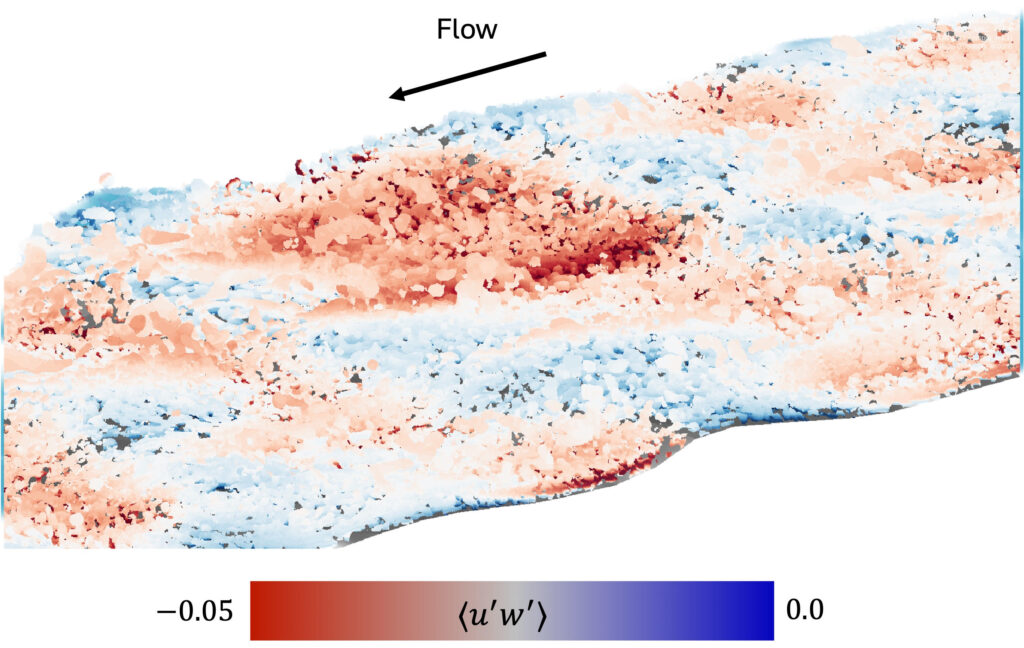
Penn Engineers have uncovered an unexpected universal pattern in how turbulent airflows behave over natural dune fields, offering insights into one of the most perplexing phenomena in fluid dynamics: the physics of roughness-driven internal boundary layers.
Internal boundary layers form when atmospheric flows encounter roughness transitions; think the interface of land and sea, the boundary of a meadow and forest, a wind farm, or tall city buildings. The inner region of the flow will gradually adjust and develop a new regime of wind velocity and turbulence in response to the dramatic roughness change. This wind pattern determines the transport of sand and dust, and the exchanges of moisture and carbon dioxide between land and the atmosphere. Modeling and predicting this behavior has been an immense challenge due to the complexity of the physics involved.
In a new paper published in the Proceedings of the National Academy of Sciences (PNAS), Justin Cooke, the paper’s lead author and doctoral student in Mechanical Engineering and Applied Mechanics (MEAM) and George I. Park, Assistant Professor in MEAM, discovered that regardless of the dune field geometry, inflow conditions, or streamwise position, the turbulent flow structures within the internal boundary layer exhibit a universal pattern when scaled by the local boundary layer height.
Their work represents an interdisciplinary collaboration combining Cooke’s expertise in turbulence modeling with insights from geophysics by Douglas Jerolmack, Professor in MEAM and in Earth and Environmental Science, who is interested in how wind and water drive the formation of landscape patterns over long timescales. Cooke and Park were able to simulate the full 3D airflow over a dune field geometry matched to the White Sands desert in New Mexico. Their simulations provide the most comprehensive picture yet of how airflow patterns evolve and interact with a full dune field topography.
Jerolmack’s team had previously conducted more than a decade of field work that documented dune dynamics, sand transport, and vertical profiles of wind velocity at White Sands. These data led to the hypothesis that the abrupt roughness transition, from salt flat to dunes, caused large-scale changes in wind flow that forced the dunes to slow down across the 10-kilometer long dune field. “We were never able to see these changes in the wind, however; we could only infer them by their consequences on the landscape,” says Jerolmack. “It’s impossible to collect the 4D (3D + time) wind data needed. What my collaborators have done allows me to actually see a pattern that up to now has only lived in my imagination.”
“This study allows us to fully visualize and quantify the detailed wind patterns and forces involved,” explains Park. The researchers leveraged extreme-scale computational fluid dynamics simulations, computing the airflow over a real dune field geometry from White Sands, New Mexico using an 86 million grid point mesh on thousands of processors. The simulations required thousands of processors over 2-3 months using the large-eddy simulation solver, CharLES. This cutting-edge flow solver was developed from a Stanford-originated technology firm (Cascade Technologies), which is now a part of Cadence Design Systems. Cooke spent significant time iterating on mesh generation, validating inflow conditions against field data measured by Jerolmack’s team, implementing turbulence models, and visualizing the simulated flows.
This story was written by Claire Sibley. To read the full article, please visit MEAM.