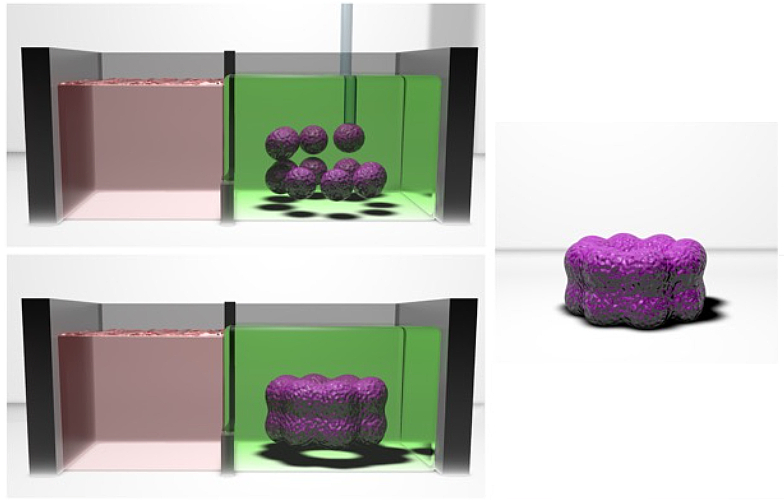
A typical 3D printer works by depositing melted plastic in layers, gradually building up a solid shape as those layers harden. Bioprinters work in a similar way, except they use living cells, which grow into rudimentary tissues much like they would in an organism.
Bioprinting is currently used to generate model tissues for research and has potential applications in regenerative medicine. Existing bioprinting techniques rely on printing cells embedded in hydrogels, which results in low-cell-density constructs that are well below what is required to grow functional tissues. Maneuvering different kinds of cells into position to replicate the complex makeup of an organ, particularly at organ-like cell densities, is still beyond their capabilities.
Now, researchers at the University of Pennsylvania’s School of Engineering and Applied Science have demonstrated a new bioprinting technique that enables the bioprinting of spatially complex, high-cell-density tissues.
Using a self-healing hydrogel that allows dense clusters of cells to be picked and placed in a three-dimensional suspension, the researchers constructed a model of heart tissue that featured a mix of cells that mimic the results of a heart attack.
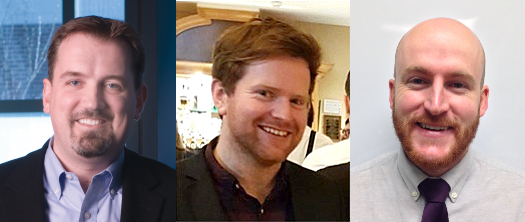
The study was led by Jason Burdick, Robert D. Bent Professor in the Department of Bioengineering, and Andrew C. Daly, a postdoctoral researcher in his lab. Fellow Burdick lab postdoc Matthew Davidson also contributed to the study, which has been published in the journal Nature Communications.
Even without a bioprinter, groups of cells can be made to clump into larger aggregates, known as spheroids. For Burdick and colleagues, these spheroids represented a potential building block for a better approach to bioprinting.
“Spheroids are often useful for studying biological questions that rely on the cells’ 3D microenvironments or in the construction of new tissues,” says Burdick. “However, we’d like to produce even higher levels of organization by ‘printing’ different kinds of spheroids in specific arrangements and have them fuse together into structurally complex microtissues.”
There are several current techniques for producing such complex structures out of spheroids, but they either damage the spheroids during processing or involve a series of lengthy and tedious steps.
Here, the research team’s advance hinged on the use of a self-healing hydrogel, which serves as a scaffolding for spheroids as they are picked and placed into the desired 3D arrangement within the hydrogel by way of a micropipette.
“Since the hydrogel reforms itself as new spheroids are dragged into position, sophisticated structures can be built up in three dimensions, as if their components were suspended in midair,” says Daly. “Furthermore, the hydrogel can be washed away after the spheroids fuse into the microtissue.”
Burdick’s group is particularly interested in modeling cardiac tissue that has experienced scarring as the result of a heart attack. Following an attack, the beating of healthy heart cells is impeded by stiffer scar tissue, reducing the organ’s overall function. Using this new bioprinting technique, spheroids with the properties of both healthy and scarred cardiac tissue can be precisely arranged into realistic 3D geometries.
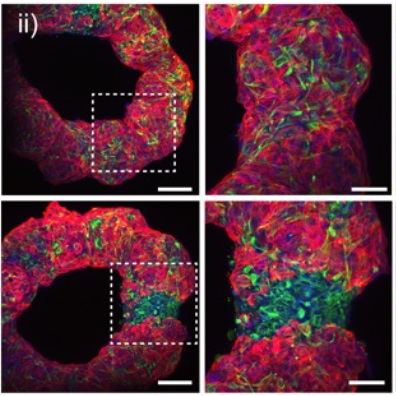
The researchers tested their technique by building rings of cardiac tissue that mimicked heart chambers with different arrangements of healthy and scarred regions, like those that might be found after a heart attack. These regions are a prime target for drugs that might be able to regenerate healthy cells, so developing tissue models that capture such features would make for better testing.
“The heterogeneity of tissues is important in the assessment of biological questions or drug screening, as it would better mimic the actual tissue response,” says Burdick. “In our experiments, we used microRNA therapeutics to regenerate cardiomyocytes, important cells that are lost after a heart attack, in the scarred regions. Testing functional outcomes, such as the electrical conductivity across the scar, would not be possible with previous approaches to bioprinting.”
Future research on the new bioprinting system will involve ways of automating, scaling and speeding up the printing process.
The research was supported by postdoctoral fellowships through the American Heart Association (20POST35210923) and the National Institutes of Health (F32 DK117568), as well as additional funding through Penn’s Center for Engineering MechanoBiology via National Science Foundation Science and Technology Center grant (CMMI: 15-48571) and the National Institutes of Health (R01HL137365, R01HL135090).