Penn Engineers’ Liquid Crystal Force Fields Enable New Kind of Microrobotics
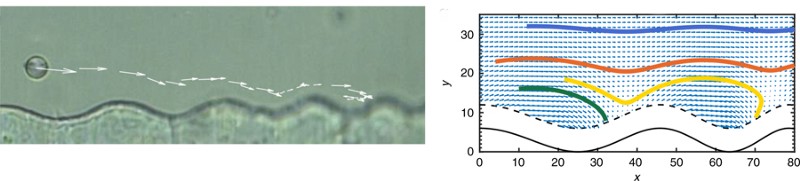
The fields of self- and directed-assembly are all about getting microscopic particles to go to predetermined locations, where they can deliver a payload, sense their surroundings or be part of a larger structure. By manipulating energy gradients in the particles’ medium, researchers are developing new ways to get them to hit their marks and stay.
These techniques have mostly focused on getting particles to form structures in response to applied fields, but new research by engineers at the University of Pennsylvania builds upon that work, suggesting that such techniques can be extended into crafting dynamic, tunable paths and particle docking sites.
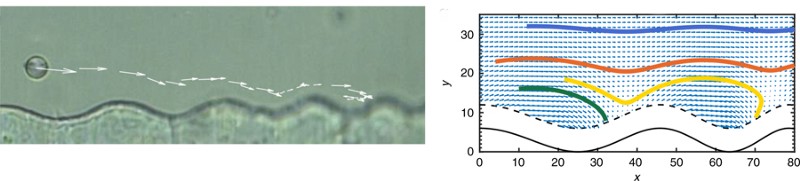
A new study, published in Nature Communications, shows how simple glass particles can be instructed to follow sophisticated trajectories, which arise from their interactions with a liquid crystalline environment.
Though the particles in question have no moving parts or internal energy sources, they can be controlled much like robots. And because this ability doesn’t stem from the particles’ chemical composition or physical properties, the same principles could be applied in a variety of applications, using particles with sensing or optical properties.
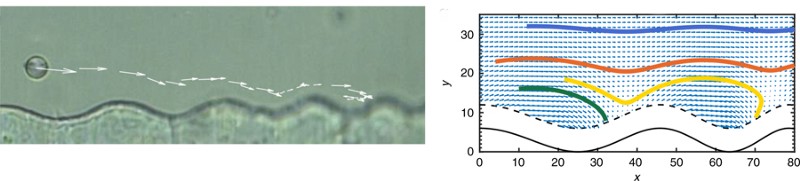
The study was led by Kathleen Stebe, Richer & Elizabeth Goodwin Professor in the Department of Chemical and Biomolecular Engineering, former Stebe lab member Francesca Serra, who is now on the faculty at Johns Hopkins University, and Yimin Luo, who recently defended her doctoral thesis. Current postdoctoral researcher Giuseppe Boniello and collaborator Daniel A. Beller from University of California, Merced also contributed to the study.
The experimental system involves liquid-crystal-filled chambers sandwiched between two microscope cover slips. The edges of these chambers feature undulating walls with bumps and divots of varying sizes. Viewed from above, particles are attracted into the “wells” and away from the “hills,” though their behavior is governed by the elastic energy stored in the surrounding liquid crystal, rather than gravity.
“The concept is that particles make distortions in the liquid crystal around them. Something as simple as a wavy wall creates its own set of distortions. When the particle is near the wavy wall, the two distortions interact,” Stebe says. “The particles move to minimize those distortions. Effectively, the distortions define elastic forces in the liquid crystal that move the particles to lower the system energy.”
“In the past,” Luo says, “we’ve looked into this phenomenon for its equilibrium behavior, just getting particles to dock in the indentations in the wavy wall. But now we see we can tune dynamics as well as equilibrium.”
The team’s work was inspired by microrobots, tiny devices that require creative approaches to sensing and propulsion so they can navigate their environments despite major constraints on batteries, motors and locomotion at that length scale.
“In microrobotics, it is important to control a robot’s path,” Stebe says. “Understanding the forces on the robot is a first step in developing means to control what it does. In the case of our liquid crystal distortions, we know where the particles are attracted and where they are repelled.”
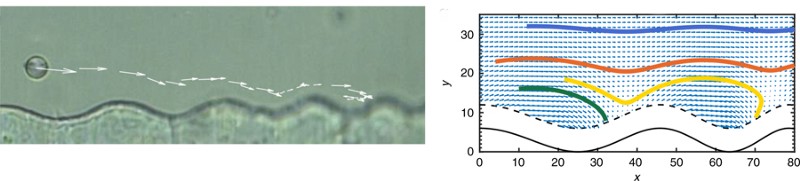
“Understanding where they are repelled ended up being incredibly powerful,” Luo says. “Particles nestle in the wells and are repelled by the hills. But depending on where they’re positioned above the hill, they’ll go on very different trajectories.”
The researchers demonstrated how the shapes of the interacting distortions influence the path the particle takes when it is repelled from a hill.
“We can drag a particle to that location with a magnetic field and let it go, and it will get propelled away from the wall by the elastic energy stored in the liquid crystal,” Stebe says. “And that path is something we can define; we can take a particle from one location and shoot it to another.”
Potential applications for this phenomenon could include reconfigurable microdevices, responsive sensors and information storage systems.
The research was supported by the U. S. Army Research Laboratory and the U.S. Army Research Office under Grant W911NF1610288.