Penn Engineers Discover New Cellular ‘Elevator’ That’s Controlled by Light
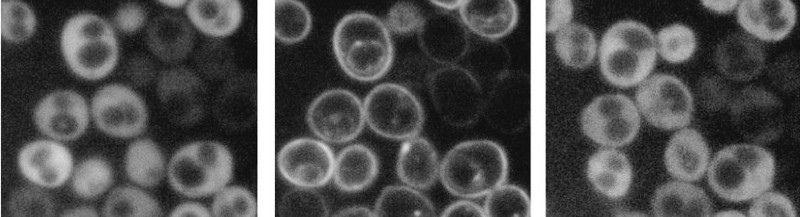
Bioengineers in the field of optogenetics are perpetually hunting for cellular mechanisms that are controlled by light. Beyond understanding the fundamentals of how photoreceptors change cell physiology in response to light, these mechanisms can ultimately be repurposed and genetically engineered into biological switches, allowing cellular behavior to be controlled with the pulse of a laser.
In a paper published in the Proceedings of the National Academy of Sciences, researchers from the University of Pennsylvania’s School of Engineering and Applied Science have demonstrated a never-before-seen behavior in a natural photoreceptor protein. When this photoreceptor is exposed to light, it moves itself from the inside of the cell to the surface, like a molecular “elevator,” by directly binding to the cell’s membrane.
This mechanism is the simplest of its kind yet reported. Other synthetic photoreceptors can be engineered to exhibit this type of elevator-like behavior, but they all need to bind to another engineered protein at the membrane in order to move.
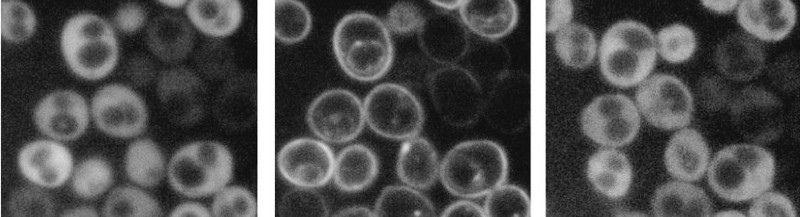
The study was led by Brian Chow, assistant professor in the Department of Bioengineering, and Spencer Glantz, then a graduate student in Chow’s group, now a postdoctoral researcher at The Jackson Laboratory. Fellow department members Erin Berlew and Benjamin Schuster contributed to the study. They collaborated with Kevin H. Gardner, Director of the CUNY Advanced Science Research Center, and Zaynab Jaber, a graduate student in his lab.
“Almost all organisms have photoreceptors in their cells, even ones that live at the bottom of the ocean, and there are many ways we know photoreceptors signal in response to light,” Chow says. “But it’s never been shown, however, that natural photoreceptors would signal in this manner, moving from the cytoplasm to the membrane by binding to membrane lipids themselves, without being recruited by another engineered protein to make the trip.”
Chow and his colleagues identified the protein from Botrytis cinerea, a pathogenic fungus that affects food crops, notably grapes and strawberries. The organism’s genome has only recently been sequenced, but their study went beyond the purely computational approach commonly used to identify potentially useful proteins, by experimentally elucidating the protein’s elevator mechanism in the lab.
“Botrytis cinerea is a difficult-to-work-with pathogen,” Glantz says, “but we managed to engineer our protein of interest in lab-friendly yeast and human cell lines instead.”
“To establish a new phenomenon like this is actually pretty rare,” Chow says. “Much of what we know in photobiology is inferred from bioinformatics, but actually proving the mechanism in cells is much, much harder. The big-data, bioinformatics approach we initially took identified about 6,000 photoreceptors in about two years, but proving the mechanism of this specific one in the lab took twice as long. It was really a herculean effort by Spencer and the others.”
The protein in question is a light-oxygen-voltage sensitive protein, or LOV, which Chow’s laboratory previously showed to be the most ubiquitous photoreceptor class found in nature.
“What’s interesting about this natural protein is that we can build on top of it by adopting it as a scaffold for future engineering. You can potentially attach whatever protein “cargo” you want to it and use the molecular elevator to shuttle that cargo back and forth in the cell,” Glantz suggests. “This naturally occurring elevator mechanism is useful to studying a variety of diseases, including cancer. When you recruit certain proteins to the membrane, you can drive cytoskeletal rearrangement in the morphology of the cell. That has a lot to do with how tumor cells proliferate and migrate, which makes them an ideal target of study.”
Chow and his colleagues are interested in applying this biological nanomachine to studying G-protein coupled receptors, or GPCRs, which are involved with signaling pathways by which cells communicate and sense their environment. Since they are found in the cell membrane, they are of particular interest to pharmaceutical applications; half of FDA-approved drugs targeting GPCRs do so in part because of the ease of delivering drugs to the cell surface compared to inside.
The group specifically intends to investigate fundamental questions of how cells encode and transfer information to one another.
“GPCRs are involved in cells’ calcium signaling pathways,” says Chow. “Calcium messengers are like electrons in a computer; calcium levels tend to oscillate, and those oscillations encode information. Cells decode these oscillations to essentially compute, for example, whether stem cells should be activated versus quiescent, or in a developing brain, which genes should be transcribed or ‘turned on.’”
The research was supported by the National Science Foundation through grants MCB 1652003, CBET 126497 and its Graduate Research Fellowship Program; the National Institute on Drug Abuse through grant R21 DA040434; the Penn Medicine Neuroscience Center; the W. W. Smith Charitable Trust for the Heart; the National Institute of Neurological Disorders and Stroke through grant R01 NS101106 and Penn Center for Neuroengineering and Therapeutics Training Grant T32 NS091006; the Defense Advanced Research Projects Agency through its Living Foundries program 5710003185; the National Institute of General Medical Sciences through grant R01 GM106239; and a National Institutes of Health postdoctoral fellowship F32GM119430.