Penn Engineers’ Gold Nanorods Key to Measuring Materials’ Squishiness at the Nanoscale
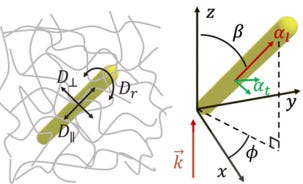
Rheology is the science of studying how soft materials and complex fluids deform and flow under stress. These materials are everywhere in biology, and since their relative stiffness or squishiness is relevant to diseases, such as cancer, there is a need to accurately measure just how squishy they are.
Researchers at the University of Pennsylvania’s School of Engineering and Applied Science have made advances in the field of “microrheology,” developing a microscopy system able to make such measurements at previously impossible length scales.
By embedding cigar-shaped gold nanorods in the material to be studied and observing them with a stereoscopic, laser-based microscope, the researchers are able to make measurements on the order of 100 nanometers or smaller. This is small enough for the researchers’ microrheology technique to be used on the membranes of cancer cells.
They plan to apply this technique to ongoing research at Penn’s Physical Sciences Oncology Center (PSOC), which aims to connect the stiffening of liver cells in cirrhosis to the progression of liver cancer.
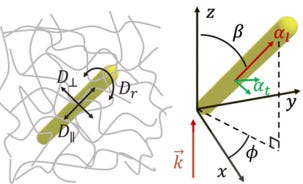
The researchers, John C. Crocker, professor in Penn Engineering’s Department of Chemical and Biomolecular Engineering, along with lab members Mehdi Molaei and Ehsan Atefi, published a study detailing this system in the journal Physical Review Letters.
“Our technique provides a unique way of probing the fluctuations and rheology of soft materials at the nanoscale for the first time,” Crocker said. “This has to potential to revolutionize experiments in soft matter and interfacial science, and provides experimental verification of dynamics that could only previously be observed in computer simulations.”
Crocker has worked on previous rheology techniques, which involve embedding microscopic spheres in the soft material, measuring their Brownian motion, and then computing the squishiness from that motion.
Brownian motion is the random movement particles in a fluid or soft material experience due to their collision with the molecules making up the material. The speed and frequency of the resulting random movement can be used to infer physical properties of that material.
Gold nanoparticles make excellent tracers, since laser-illuminated microscopes can easily pick them out of the material they are suspended in, and spherical nanoparticles are relatively easy to make. However, the geometry of a sphere has an intrinsic limitation: it looks the same from all angles.
“Tracking rotation can give us a higher resolution than just tracking translation, or side-to-side, up-or-down movement. Brownian motion does cause spheres to rotate, but you can’t visually tell that they have,” Molaei said. “Using nanorods instead of spheres makes our technique more sensitive and enables it to work in stiffer materials, where the motion is relatively small.”
The researchers tested their technique on a model polymer with well-understood rheological properties, using a laser-illuminated dark-field microscope with two different polarizations to track the nanorods. Somewhat like a 3D movie, contrasting the data from the two polarizations allowed the researchers to calculate the rods’ orientation in space.
“The result turns out to be orders of magnitude superior to previous methods in several important metrics, including working on volumes of goo as small as an attoliter, or a quadrillionth of a milliliter.” Crocker said. “Still, the rods are way smaller than what can actually be resolved in an optical microscope; thousands could fit inside a single E. Coli. We had to solve a lot of optics calculations in order to quantitatively convert optical polarization to orientation.”
New research in collaboration with PSOC Director Dennis Discher, Robert D. Bent Professor of Chemical and Bimolecular Engineering, is already underway. Molaei is measuring the stiffness of liver cell membranes in vitro.
“The idea is that the membrane has waves and undulations in it that depend on the membrane’s mechanical state, as well as the proteins in it, and the proteins it’s attached to,” Molaei says. “Our collaborators at the PSOC thinks that part of liver cancer relates to how cells sense the stiffness of the liver, since cancer is almost always preceded by cirrhosis, but how they do that sensing is poorly understood. These membrane undulations might be the answer, but they have not been measured before.”
The research was supported by the American Chemical Society through its Petroleum Research Fund Award #55120-NDS, and by Penn’s Physical Sciences Oncology Center.