It’s a Small World
A Conversation with Danielle Bassett and Duncan Watts
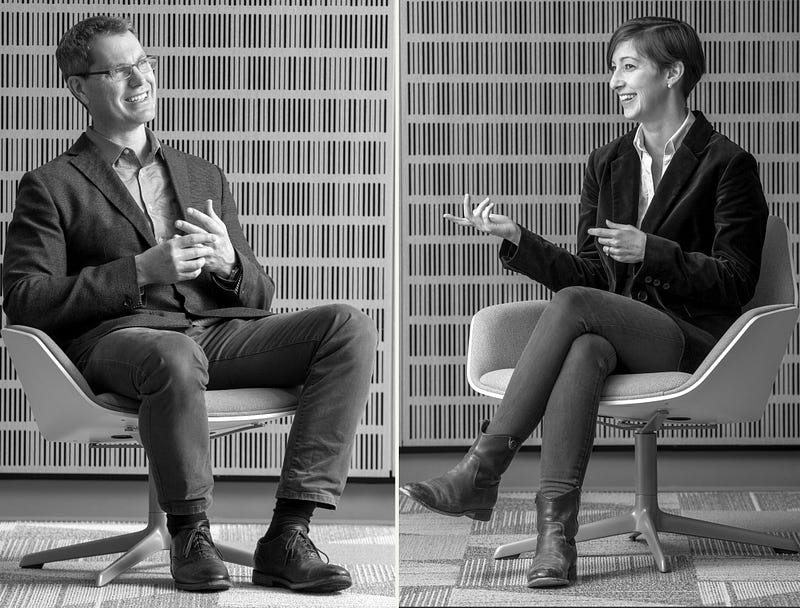
In July, Duncan Watts joined Penn Engineering as the University’s 23rd Penn Integrates Knowledge Professor. As such, Watts will have primary appointments in the Department of Computer and Information Science in Penn Engineering, as well as in The Wharton School and the Annenberg School for Communication. His research reflects the value of these kinds of interdisciplinary connections; he is one of the progenitors of the field of network science, which studies how unique members of dynamic systems interact to influence the behavior of the whole. Watts sat down with Danielle Bassett, Eduardo D. Glandt Faculty Fellow and Associate Professor in the Departments of Bioengineering and in Electrical and Systems Engineering in Penn Engineering. Bassett’s own work on network science draws from the worlds of physics, electrical engineering, applied math and neuroscience, as well as Watts’ theories. The two discussed their intertwining academic networks and how combining basic and applied science contributes to the culture of innovation at Penn. An excerpt of their conversation follows.
Danielle Bassett (DB): I came into the field about a decade after you, so I was influenced by your work. But even in the mid-2000s, the term “network science” was not used that frequently. What was it like when you were getting your start?
Duncan Watts (DW): Network science definitely didn’t exist when I started doing network science.
There were little pockets of other disciplines, like with graph theory in mathematics, and sociology and anthropology with social network analysis. But there wasn’t any field that thought about the network as an organizing principle across different areas of applications.
That, to me, is what network science is — this idea that lots of problems of interest across many disciplines can be viewed through the lens of a network. You have some collection of entities that are connected to one another, and the structure of that network could have important consequences for the behavior of the whole system.
That was the idea that Steven Strogatz [Jacob Gould Schurman Professor of Applied Mathematics at Cornell University] and I were working from — this notion that you should be thinking about the structure of the network to understand the dynamics of the system — in our paper, “Collective Dynamics of Small World Networks” in 1998. But it wasn’t actually called network science until several years later.
DB: As it happens, my first paper was called “Small-world Brain Networks,” and I finally have a paper coming out with Strogatz as a coauthor, so now you and I are separated only by two steps in the worldwide co-authorship network.
That notion of “small worldness” was very important and has continued to be very important for a really long time. For many physical systems, like a crystal structure for example, we can sort of blur our eyes and say that, even though there are lots and lots of atoms, they roughly interact with each other in the same way.
But with small-world networks, we’re increasingly realizing there are many systems where that kind of assumption is completely false. It requires that we understand the underlying architecture of these interactions in order for us to see how the system will behave.
DW: When we first started tackling this problem, we were thinking about a biological question — the synchronization of crickets — from a mathematical modeling perspective. The realization that I had at the time was exactly what you just said. All of the models used this “mean field assumption” where everything is interacting with the average of everything else. And that seemed completely false in the context of crickets sitting in trees chirping at each other.
So I was mulling over what would be a framework for describing the actual system. And I recalled a conversation I had with my father about a year earlier when he casually mentioned that you’re only ever six handshakes away from the president of the United States. I hadn’t heard that at the time, but later I learned that this was a topic that had come up over the years in sociology, called the small-world problem — also known as six degrees of separation.
DB: I’m also very curious about how small teams can come up with very unusual and creative ideas, and which group of people is best at doing that. It’s hard to predict the way people interact that allows that to happen.
DW: Well, that’s a good question, and I think it’s relevant to academia. A couple of years ago I wrote a paper about what I called solution-oriented social science. If you pick any kind of nontrivial problem in social science, psychologists will have written about it and sociologists will have written about it and political scientists will have written about it. You end up with 5 or 50 perspectives that are so different from one another that you cannot figure out how they all fit together.
After a while it occurred to me that this was really a consequence of the way that academic research is organized. We are all trained within a particular discipline, and our individual toolboxes produce such different ways of thinking that it’s not even as simple as deciding which one’s answers are right or wrong. They’re not even necessarily comparable.
I think the myth that we have about the academic publishing system is that it somehow sorts this problem out. That everybody publishes their papers and then we all read each other’s papers and then, over time, knowledge will accumulate. And I think actually that doesn’t really happen very much, and it especially doesn’t happen across disciplines.
The approach I’m interested in, and this is somewhat inspired from having worked in an industry lab for the last decade or so, is that rather than starting with a theory and asking, “What does my theory have to say about problem x?”, you ask, “What is the theory we need to build in order to solve this problem?”
DB: It struck me that the goal that you’re outlining is actually very similar to the way science was done 2,000 years ago. These ancient philosophers were looking at the world and saying, “Oh, that’s interesting, I wonder why…” And they were doing a lot of characterization, but were also building theory from observations of a real system. It made me wonder whether we’ve digressed by siloing the disciplines and training students to have a narrow view of what science is.
DW: You can go back to the ancients, but a lot of this specialization has really happened in the last hundred years.
One of the turning points was a very influential memo written by Vannevar Bush, who was the director of the Office of Scientific Research and Development under Franklin Roosevelt and the godfather of the National Science Foundation. He described this model of science as moving in a linear path from basic to applied science. Bush felt very strongly that the two shouldn’t mix, but this was always a bit of a myth — it didn’t really come from anywhere except Bush’s impression of how things should work.
DB: I’ve been talking to my department chair about developing a broad course that asks students to think carefully about those questions — the definition of science, its history and its goals. What would you put on the syllabus?
DW: About 20-odd years ago, there was a really nice book written by Donald Stokes called “Pasteur’s Quadrant.” He pointed out that what Bush had described was incomplete; at minimum, you should think of science along two axes. One axis would be to what extent it is advancing basic understanding and on the other axis should be whether it is related to some sort of application.
You have traditional basic science, which is curiosity-driven and has no obvious application, in Bohr’s quadrant, which is in reference to Niels Bohr’s theory of the atom. And then you have applied science, which is about trying things until they work and not interested in advancing basic understanding, in Edison’s quadrant. But then there was Pasteur’s quadrant, where it’s both things at the same time.
You are solving an applied problem, but you are also advancing basic understanding at the same time. And by the way, Ben Franklin, when he wrote the mission statement of the University of Pennsylvania, specifically stated that the purpose of a university was not just to develop basic knowledge but also to solve applied problems. Penn is really a good place to be doing this.