The first-ever image of a black hole, published in 2019, was constructed from five petabytes of data. These files were so large that they had to be flown to data analysts in giant stacks of hard drives; a plane ride from Hawaii was faster than the internet. Thankfully, Firooz Aflatouni is improving the fundamentals of data transfer to make sharing information about black holes — or just texting a friend — a faster, more efficient process.
Aflatouni, Skirkanich Assistant Professor in Electrical and Systems Engineering, has built his career on designing clever combinations of electronic and photonic technology with applications from laser-based 3D imaging, to microwave “cameras” that can see through walls or into tumors, to brain-machine interface systems. One current project, funded by the Defense Advanced Research Projects Agency (DARPA), focuses on making an electronic-photonic chip that can transfer tens of thousands of times more data per second than those found in current modems.
Right now, many systems are switching from sending information electronically to using fiber optics, which encodes information in the amplitude of light waves. Because light waves have a much higher frequency than electrical signals, fiber optic cabling is already making ultrafast connections between computers, and optically connected CPUs have the potential to make the computers themselves much faster. As of now, systems can’t quite make the most of this speed. Currently, there are separate chips to deal with electronic and photonic information, and communication between these chips is costly.
Transferring data from the electronic domain to the optical domain and back takes valuable time and energy. Aflatouni’s Electronic Photonic Microsystems Lab constructs chips that handle electronic and photonic information in the same place — an approach called “electronic-photonic co-design.”
“Our system has electronic and photonic devices co-integrated next to each other on a single chip,” says Aflatouni. “Electronic circuits control the optical devices and take massive amounts of data, organize it in a certain fashion and place it on optical signals. But it’s all done on the same chip.”
Implementing the entire system on a single chip through electronic-photonic co-design enables data transfer at a very high rate while using less space and energy than systems that require multiple electronic and photonic chips with complex chip-tochip connections.
For example, the newest televisions rely on fast fiber optic networks to stream content in “8K” resolution; if those enormous files were to come in through cable boxes outfitted with Aflatouni’s technology, no energy would be wasted juggling data between different chips. For the satellite communication that many systems depend on, Aflatouni has a similar electronicphotonic co-design solution. While microwave signals are typically used for satellite communications, in a project funded by NASA, Aflatouni is replacing these microwave signals with laser beams. By switching from microwaves to light waves, more information can be transferred per second using much less energy.
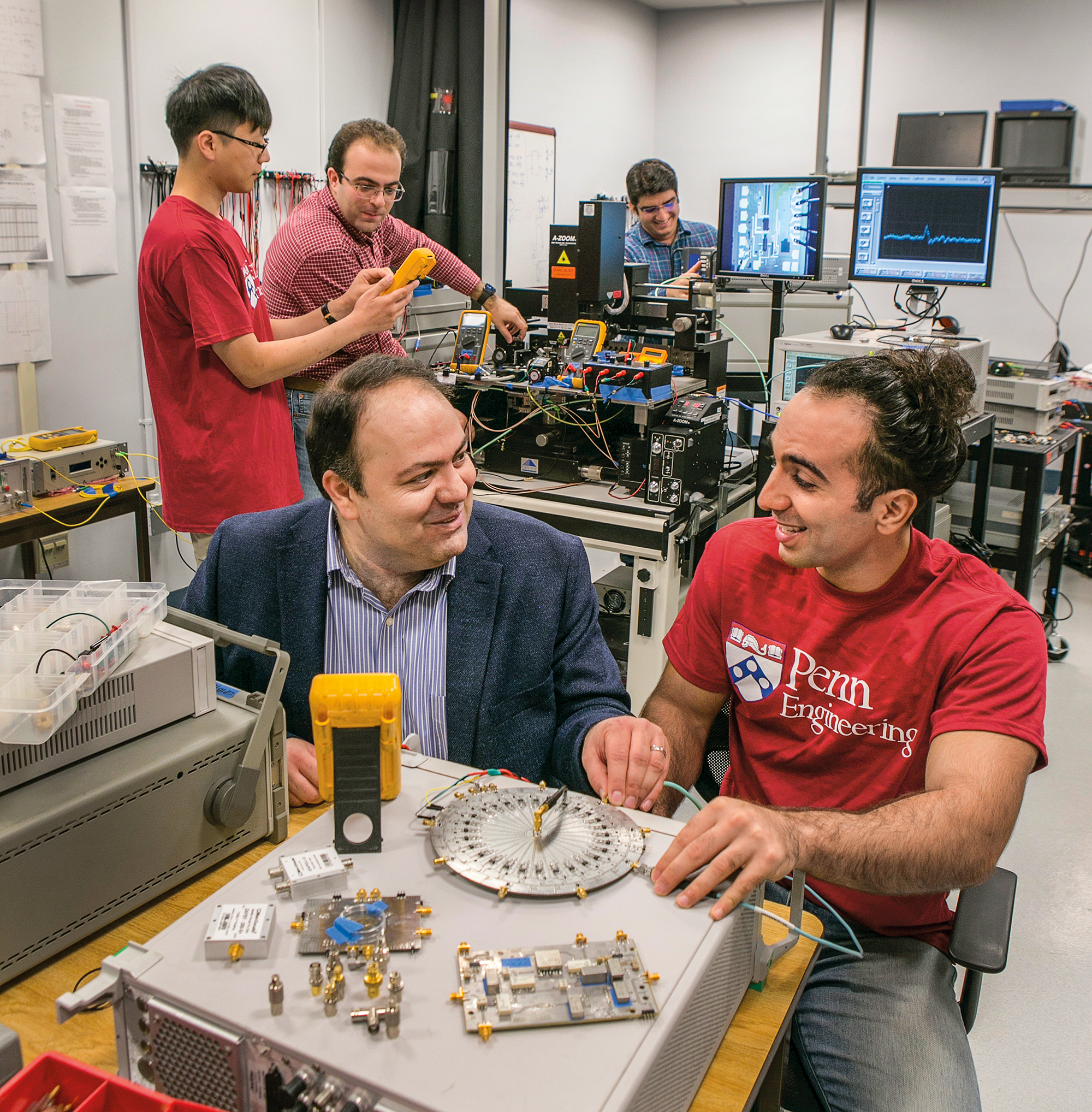
Drowning in Data
When it comes to limitations in transfer speed, astrophysics isn’t the only field struggling. The human brain, for example, contains approximately 75 billion neurons, each of which could be firing at any given millisecond. With currently available technology, large-scale neural recordings produce more data than is possible to gather and transfer.
When collaboration opportunities with researchers in biology arose, Aflatouni recognized the interdisciplinary potential of his work. For one project, he is working to make a chip that can record neural activity, classify the recorded electrical pulses and transfer that data while consuming very little energy. In the future, this low-energy, implantable brain monitoring system could help diagnose and control certain neurological disorders such as epilepsy.
Other examples of Aflatouni stepping outside of his engineering wheelhouse include designing electronic and photonic chips to detect hint traces of biological indicators of disease in blood, a handheld microwave imaging system that can see through walls or skin, a high-frequency droneto-drone communication system, and electronic control chips for quantum computing.
“I’m generally open to pursuing ‘out-there’ opportunities, so my work builds off of my core electronic-photonic area and is pretty diverse. My research has benefitted significantly from Penn’s culture of collaboration,” says Aflatouni.
Industry to Academia
Being able to collaborate and step outside of his comfort zone is part of what drew Aflatouni away from industry and into academia. Before embarking down the path to his doctorate and current position at Penn, Aflatouni was fully immersed in the business world. After graduating from college, he started his own company, where he served as the CTO for six years.
As an undergraduate student in Tehran, Aflatouni was already thinking about emerging problems in the satellite communications that he’s currently transforming with lasers. These satellites rely on two main mechanisms to function in space: The first is their capacity to relay data using microwave signals and the second is their ability to maintain a geostationary orbit, meaning their position appears fixed relative to a reference point on Earth. The former ability is powered by solar panels, but the latter is enabled by thrusters with a limited amount of fuel. When this fuel runs out, satellites enter an “inclined” orbit that wobbles through the sky. This means that signals from that fixed reference point no longer hit their target.
Aflatouni’s startup sought to fix this problem by creating an affordable satellite tracking system. His company’s system allowed reliable, uninterrupted communication once a satellite started wobbling, meaning that companies kept turning a profit and customers’ technology kept working. But eventually, Aflatouni left industry to pursue a doctoral degree.
“One of the reasons that I moved from industry to academia is that I felt kind of limited. Especially as a startup, if you try something new and it doesn’t work, you may lose the market,” says Aflatouni. “In academia, the tolerance for uncertainty is much higher. You can try riskier ideas.”
Knowledge Transfer
Growing up, Aflatouni developed an early interest in electronics. He remembers tinkering with electronics kits that his father and sister bought him, trying to understand how devices work.
“When I was fourteen or fifteen, I built a device to voice control the lights in our house,” Aflatouni says. “Creating these sorts of devices, I got electrocuted a few times. But the first time I got a very simple electronic circuit to work, it was such a huge reward. It gave me some confidence.”
Aflatouni has passed this spark of interest down to another generation. Each summer, he collaborates with his daughters, currently six and nine years old, on an engineering project. Last summer, the family team built a robot from scratch, with Aflatouni’s older daughter picking up some basic scientific thinking as well as coding skills in the process.
From his daughters to his graduate researchers, Aflatouni likes to teach his students to keep pushing the boundaries of their knowledge. At weekly lab meetings, his students partake in the usual research updates but also in cultural presentations, where students talk about the customs, food or tourist attractions of the places they’re from, and participate in interdisciplinary science presentations.
“I want students to know what’s happening in the world, so they can identify important problems,” says Aflatouni. “We’ve had presentations about gravity wave measurements, the first image of a black hole and Amazonian ant behavior. Whatever it is, it’s not directly relevant to their research. We learn collectively. We get a chance to see problems and see if we might be able to contribute.”
This article appeared in the Fall 2019 edition of Penn Engineering Magazine.