Adding a Twist Makes 3D-Printed Structures Tougher
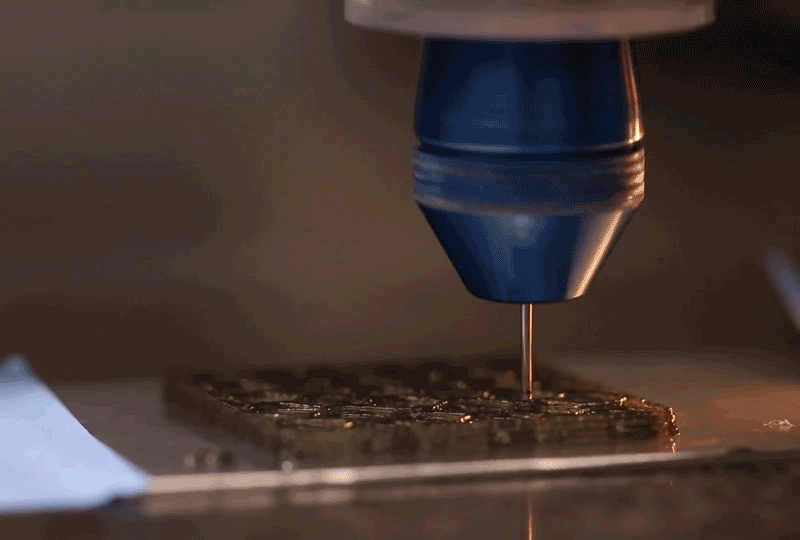
Some mantis shrimp kill their prey with a hammer-like appendage called a dactyl club. Despite being an organism only a few inches long and weighing about as much as a deck of playing cards, the shrimp can throw shell-shattering punches at more than 50 miles per hour.
If human engineers were to design a hammer that was simultaneously so light and so tough, they might look to relatively rare combinations of elements, like titanium alloys, but the shrimp’s club is made of not much more than carbon and calcium. But while the elemental palette is simple, the way in which the elements are arranged at the microstructure is far more complex than scientists and engineers can produce in synthetic materials. Thanks to complicated microscopic structural arrangements, mantis shrimp can repeatedly use their clubs without breaking them or hurting themselves.
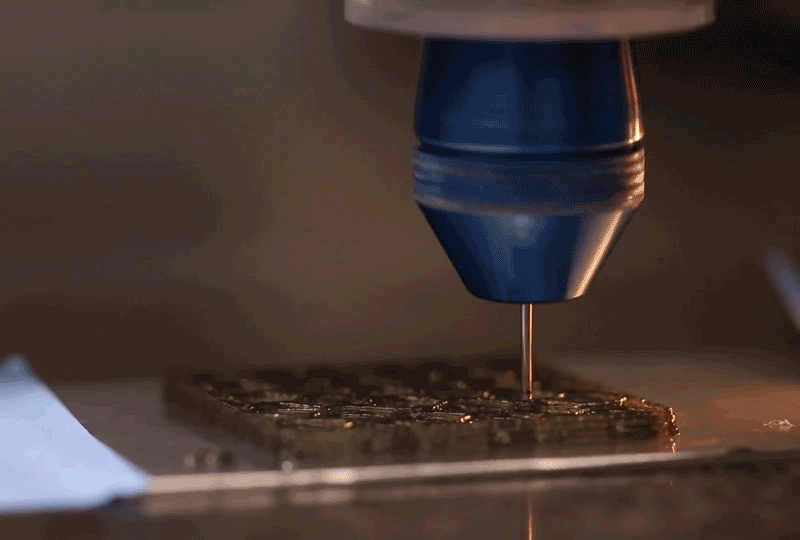
Jordan Raney, assistant professor of Mechanical Engineering and Applied Mechanics, has helped devise a way for 3D printers to make structures with similar toughness-increasing patterns embedded within.
By infusing liquid polymer “ink” with short carbon fibers and rotating the printer’s nozzle as it prints, the fibers twist into helical microstructures akin to those found in the mantis shrimp’s club. Changing the timing and speed of rotation will allow designers to exercise finer control over the internal composition of their prints, even when working at large scales.
Raney is a lead author of a paper describing this technique, published in the Proceedings of the National Academy of Sciences. He conducted the research while a postdoctoral researcher in the lab of Professor Jennifer A. Lewis at Harvard University’s John A. Paulson School of Engineering and Applied Sciences. The other lead author, Brett Compton, now at the University of Tennessee, Knoxville, along with visiting graduate student Jochen Mueller from Professor Kristina Shea’s lab at ETH Zurich, also contributed to the work.
Most 3D printers melt and extrude plastic; this gives designers good control over the print’s overall shape, but little control over that shape’s internal microstructure. Such prints tend to be brittle and prone to shattering, limiting their applications.
“If you want to make 3D printing into a manufacturing platform, rather than just for prototyping, you need higher quality materials,” Raney said. “We drew inspiration from nature; many natural materials are very light, but still very stiff, strong and tough, and are composed of the simplest, most abundant elements in the natural environment, such as carbon and silicon. The performance of natural materials is driven by the optimized arrangement of these, rather than through the use of exotic elements”
Marine biologists had long marveled at the mantis shrimp’s club, but the secret to its durability was only recently discovered: helical arrangements of fibers in the club’s microstructure. The density of the these spiral-shaped structures are also different at the striking surface of the club and the parts closer to the shrimp’s soft body, dissipating the force of impact so the shrimp doesn’t hurt itself with each punch.
These helical microstructures gave the team the idea to introduce carbon fibers into a liquid polymer and to twist them into helical shape as the composite material was extruded. After the material solidified, regions with the helical microstructures were much more resistant to damage than regions where the fibers were aligned in parallel.
“Cracks tend to want to follow the fibers, so if the fibers are all straight and aligned, cracks can propagate very easily,” Raney said. “Natural materials, like the chitin in the shrimp’s club, tend to have complicated fiber arrangements with lots of variations in their angles from location to location, which means there’s a higher energy cost for cracks to grow.”
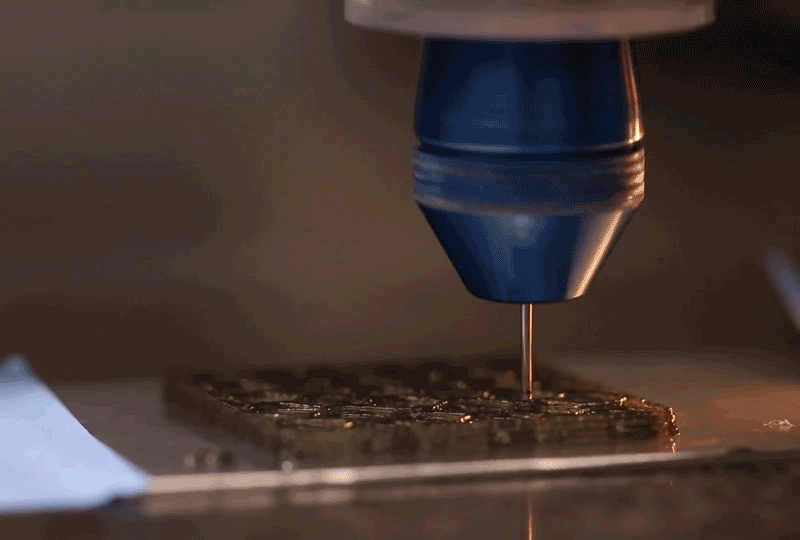
The ability to change the rotation of the nozzle on the fly is also critical, as it enables different regions of a print to be imbued with different structural properties. Adding that level of detail using existing 3D-printing technology was possible, but it came with tradeoffs: the smaller the level of detail, the smaller the maximum size of the print.
“In all of 3D printing, you’re always limited by your length scale. You can typically maximize your build volume or your precision, but not both at once,” Raney said. “One of the added strengths of this approach is that we’re starting to get around that limitation. The nozzle’s diameter still determines our overall volume, but since it’s also extruding internal fibers, we’re patterning structure at a smaller length scale at the same time.”
Harvard’s press release on the paper is available here.