As our ability to manipulate materials on the nanoscale grows, our library of “two dimensional” materials increases. Consisting of a repeating lattice of atoms in a single layer, 2D materials have unique, valuable properties that stem from being all surface. Fully understanding these properties, however, is often harder than crafting the materials themselves.
A team of engineers and physicists from the University of Pennsylvania and the University of California, Merced have now discovered a new mechanism that controls their friction behavior at the nanoscale.
In a study published in the journal ACS Nano, the team found that the presence of larger atoms within the lattice of a 2D material unexpectedly decreased the friction encountered by the tip of an atomic force microscope probe as it slid along the surface.
Understanding the interplay between the sizes of individual atoms and the spacing of the lattice they produce will be critical in tailoring the properties of new 2D materials.
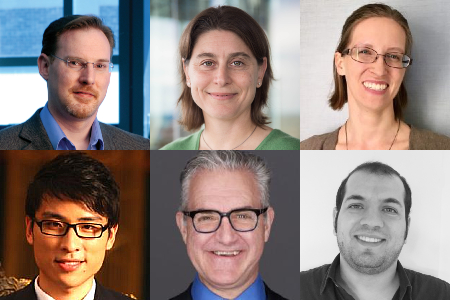
The study was led by Robert Carpick, John Henry Towne Professor in Penn Engineering’s Department of Mechanical Engineering and Applied Mechanics, and Ashlie Martini, Professor of Mechanical Engineering at UC Merced, along with Kathryn Hasz and Mohammad Vazirisereshk, graduate students in their respective labs. They collaborated with A. T. Charlie Johnson, Rebecca W Bushnell Professor in Penn Arts & Sciences’ Department of Physics and Astronomy, and Mengqiang Zhao, a postdoctoral researcher in his lab. Hasz is now a postdoctoral researcher at the University of Colorado, Boulder; Zhao is now a faculty member at the New Jersey Institute of Technology.
In their study, the researchers compared three 2D materials from the same family, transition-metal dichalcogenides (TMD). These materials consist of a metal atom, molybdenum (Mo), bonded to two atoms of one of the chalcogens, the group of elements that include sulfur (S), selenium (Se) and tellurium (Te). Because these elements are all in the same column of the periodic table, they bond equally well with molybdenum and can make 2D lattices with the same overall pattern under carefully controlled conditions.
In its common form as a thick coating, a powder or a suspension, MoS2 is a ubiquitous industrial lubricant, so the possibility of increasing its performance has spurred research into advanced synthesis and manufacturing techniques for it and other TMDs. Some of Carpick and Martini’s own research has been motivated by a desire to better understand the dynamics that govern friction when such materials are in their 2D form, and when their composition is altered.
In their new study, the researchers pursued this investigation by testing other 2D materials in the TMD family.
The researchers scanned samples of MoS2, MoSe2 and MoTe2 with the tip of an atomic force microscope probe, which works like a record player needle to measure the resistance it encounters as it slides along a surface. The experiments were complemented by atomic-scale modeling of the probe-surface interactions.
Based on prior computational results, the researchers expected that selenium and tellurium atoms, being larger than sulfur atoms, would present larger energy barriers for the tip to overcome and thus increase the overall amount of friction. In other words, larger atoms means a “bumpier” road, and thus more friction encountered to move across it
That expectation was proven to be incorrect. “Other researchers predicted that friction would go up as the size of the chalcogen increased,” says Martini. “But our results show that it goes in the opposite direction, because the larger chalcogen makes the lattice spacing larger.”
“It’s like kicking a field goal in football,” says Carpick. “Everyone thought we could make it easier to ‘score’ by lowering the goal posts, that is, lowering those energy barriers by reducing the size of the atoms.
“Here, however, we made the goal posts wider,” he says. “As we tested materials with progressively bigger atoms, they those atoms were progressively farther apart. Sure enough, we get through the goal posts more easily.”
This study not only revealed a unexpected friction mechanism, but showed the potential for entirely novel 2D materials — beyond MoS2 and graphene — for low friction applications.
This work was supported by the National Science Foundation (NSF) through awards CMMI-1762384, CMMI-1761874, and MRSEC DMR-1720530. This work used the Extreme Science and Engineering Discovery Environment (XSEDE), which is supported by NSF grant ACI-1548562.