As renewable sources such as wind and solar are quickly changing the energy landscape, scientists are looking for ways to better store energy for when it’s needed. Fuel cells, which convert chemical energy into electrical power, are one possible solution for long-term energy storage, and could someday be used to power trucks and cars without burning fuel. But before fuel cells can be widely used, chemists and engineers need to find ways to make this technology more cost-effective and stable.
A new study from the lab of Penn Integrates Knowledge Professor Christopher Murray, led by graduate student Jennifer Lee, shows how custom-designed nanomaterials can be used to address these challenges. In ACS Applied Materials & Interfaces, researchers show how a fuel cell can be built from cheaper, more widely available metals using an atomic-level design that also gives the material long-term stability. Former post-doc Davit Jishkariani and former students Yingrui Zhao and Stan Najmr, current student Daniel Rosen, and professors James Kikkawa and Eric Stach, also contributed to this work.
The chemical reaction that powers a fuel cell relies on two electrodes, a negative anode and a positive cathode, separated by an electrolyte, a substance that allows the ions to move. When fuel enters the anode, a catalyst separates molecules into protons and electrons, with the latter traveling toward the cathode and creating an electric current.
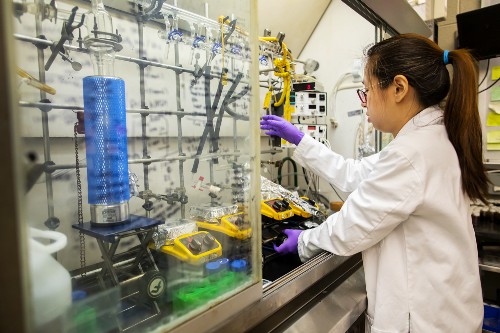
Catalysts are typically made of precious metals, like platinum, but because the chemical reactions only occur on the surface of the material, any atoms that are not presented on the surface of the material are wasted. It’s also important for catalysts to be stable for months and years because fuel cells are very difficult to replace.
Chemists can address these two problems by designing custom nanomaterials that have platinum at the surface while using more common metals, such as cobalt, in the bulk to provide stability. The Murray group excels at creating well-controlled nanomaterials, known as nanocrystals, in which they can control the size, shape, and composition of any composite nanomaterial.
In this study, Lee focused on the catalyst in the cathode of a specific type of fuel cell known as a proton exchange membrane fuel cell. “The cathode is more of a problem, because the materials are either platinum or platinum-based, which are expensive and have slower reaction rates,” she says. “Designing the catalyst for the cathode is the main focus of designing a good fuel cell.”
The challenge, explains Jishkariani, was in creating a cathode in which platinum and cobalt atoms would form into a stable structure. “We know cobalt and platinum mixes well; however, if you make alloys of these two, you have added atoms of platinum and cobalt in a random order,” he says. Adding more cobalt in a random order causes it to leach out into the electrode, meaning that the fuel cell will only function for a short time.
To solve this problem, researchers designed a catalyst made of layered platinum and cobalt known as an intermetallic phase. By controlling exactly where each atom sat in the catalyst and locking the structure in place, the cathode catalyst was able to work for longer periods than when the atoms were arranged randomly. As an additional unexpected finding, the researchers found that adding more cobalt to the system led to greater efficiency, with a 1-to-1 ratio of platinum to cobalt, better than many other structures with a wide range of platinum-to-cobalt ratios.
The next step will be to test and evaluate the intermetallic material in fuel cell assemblies to make direct comparisons to commercially-available systems. The Murray group will also be working on new ways to create the intermetallic structure without high temperatures and seeing if adding additional atoms improve the catalyst’s performance.
This work required high-resolution microscopic imaging, work that Lee previously did at Brookhaven National Lab but, thanks to recent acquisitions, can now be done at Penn in the Singh Center for Nanotechnology. “Many of the high-end experiments that we would have had to travel to around the country, sometimes around the world, we can now do much closer to home,” says Murray. “The advances that we’ve brought in electron microscopy and X-ray scattering are a fantastic addition for people that work on energy conversion and catalytic studies.”
Continue reading at Penn Today.