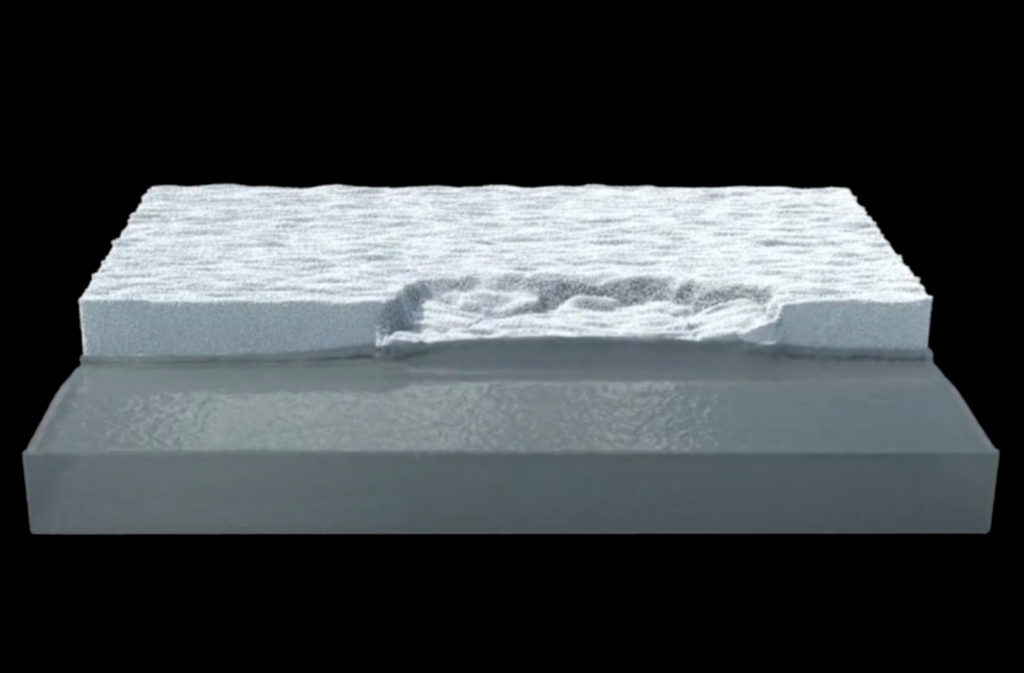
As natural disasters intensify due to climate change, accurate predictions of weather patterns and mechanisms are greatly needed to mitigate damage. Coastal regions will be the most affected by changing weather, with events such as tsunamis and hurricanes becoming more frequent and life-threatening. While most tsunamis are caused by earthquakes and tectonic activity, the warming of the planet is now increasing the occurrence of tsunamis caused by glacier calving, when chunks of glacier break off and become icebergs. Additionally, glacier calving is predicted to be the main contributor to sea level rise in the near future.
Understanding the mechanisms of large-scale ice fall is therefore crucial for mitigating a range of climate change consequences, and thanks to a new study led by researchers at the University of Pennsylvania’s School of Engineering and Applied Science, there is now a computer model that can accurately simulate those dynamics.
The interaction of ice and water with respect to gravity and buoyancy is complex, and modeling both phenomena requires an integrated approach. The study, published in the recently established journal Nature Communications Earth & Environment, describes a new simulation by Penn Engineers that models three key aspects: the ice fracture mechanics within the glacier, the fluid dynamics of the surrounding ocean water, and the interaction between the two.
The researchers employed a technique known as the material point method (MPM) which is used for a simulating the interactions between matter of different phases. Their study shows that this technique, responsible for the hyper-realistic snow in movies such as Frozen, is capable of accurately describing glacial calving and resulting tsunami waves.
By demonstrating their model’s predictive capabilities, the researchers are refining the empirical calving laws used in large-scale earth-system models, as well as improving hazard assessments and mitigation measures in coastal regions, which are essential in the context of climate change.
The study was led by Joshuah Wolper, then a graduate student in Penn Engineering’s Department of Computer and Information Sciences (CIS) and current Postdoctoral fellow in Mechanical Engineering and Applied Mechanics (MEAM), Chenfanfu Jiang, then assistant professor in CIS, now an assistant professor in the Department of Mathematics at UCLA, and Professor Johan Gaume, head of SLAB, the EPFL Snow and Avalanche Simulation Laboratory in Switzerland.
The international team of co-authors included Ming Gao, then a member of Jiang’s lab at Penn Engineering, Professor Martin Lüthi of the Department of Geography at the University of Zürich, Valentin Heller, Assistant Professor in hydraulics at the University of Nottingham, and Andreas Vieli, Professor in glaciology at the University of Zürich.
Prior to this study, glacial calving models were simplified and limited to single calving events, and do not consider the effect of the interaction between ice and water as tsunami waves, limiting their ability to predict larger scale impacts of ice fall. Likewise, most models of tsunami waves have been generated from observing landslides, with only a few based on the effects of glacial calving.
Results from the models of tsunami waves generated by glacial calving have shown that these waves are overestimated if using a landslide as the source, but a complete model that integrated glacial calving events and the subsequent creation of tsunami waves was not developed until now.
“The material point method was an exciting simulation model to apply to this problem,” Wolper says, “because materials are represented as millions of particles, each with its own evolving properties. Solving the governing physical equations enables MPM to capture large deformations and topological changes in those materials, making it ideal for representing both the fracture and solid-fluid coupling aspects of glacial calving.”
The researchers had used MPM to model avalanches in previous research; after adapting some of its equations to capture the less porous nature of ice, they were able to model how icebergs fractured off glaciers, the collision of these icebergs into the surrounding water, and how those collisions produce waves.
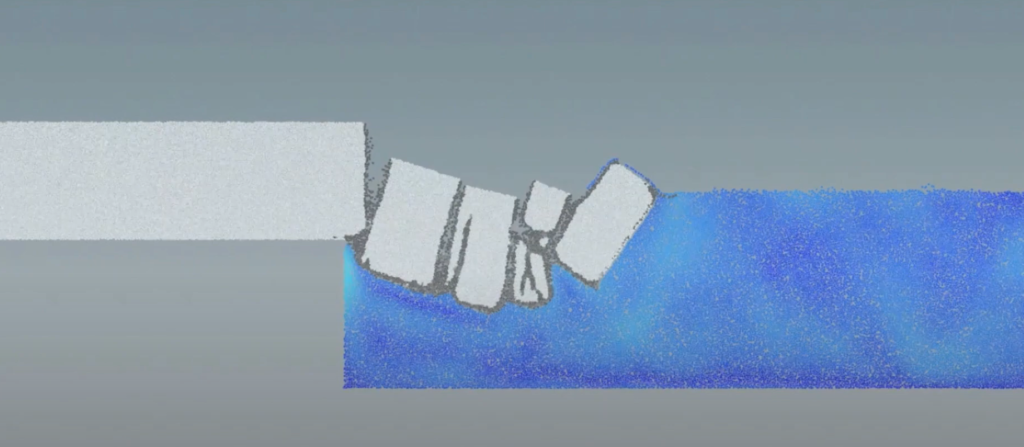
After all this computation work, however, the model still needed to be validated by observing the system in the physical world. Model validation can be done as laboratory experiments, creating representations of glacial calving, or by observing the real thing. This study included both.
“While our large-scale 3D results are exciting to look at, we still needed a robust way to validate our model in the real-world,” Wolper says. “We validate our method in three crucial ways. In the first, we compared our simulated wave speeds against those measured in a real-world experimental basin of water at the Deltares Institute in the Netherlands; we found our results to be in good agreement with this laboratory setting under a variety of ice calving mechanisms.
“Our second validation method focused on using an analytic beam bending model to validate the lengths of our fractured icebergs; we found that our model is able to predict accurate iceberg lengths for a variety of ice submergence depths, including asymptotic behavior that occurs as the ice weight and water buoyancy begin to balance out.
“Finally, the third validation method focused on a real-world calving event at Eqip-Sermia, an ocean-terminating glacier in Greenland. This event was not only captured on camera by a nearby tour boat, but additionally measured by a team of researchers using a terrestrial radar interferometer and tide gauge. We recreated this ice sheet and connecting fjord in 2D and found that the iceberg size, failure plane angle, wave amplitudes, and even the average wave speed were in good agreement with the large-scale data available from this event.”
The model was validated by comparing the characteristics and mechanisms of this real-world event, showing good agreement and accurate predictive results.
For example, the model could predict whether a given glacier would rupture closer or farther from the sea line. The height at which calving occurs is a major factor in the resulting iceberg’s size, which in turn determines the power of the wave it produces when it hits the water. The model can also predict the general shape of icebergs; top-heavy ones will rotate once submerged, leading to additional waves.
These predictions could help coastal managers defending against tsunamis, as well sea level rise and other risks stemming from climate change.
“Climate change is an inevitable reality facing us as a global community,” says Wolper. “I think the coolest takeaway from this work is that, as our computational power increases, so can our understanding of the world around us. Through the hard work of international, highly interdisciplinary teams such as ours, the research is here, and now it’s just up to us as a civilization to use it.”
The research was supported by the Swiss National Science Foundation grants PCEFP2_181227 and 200021_156098, the European Community’s Horizon2020 Research and Innovation Program through a grant to HYDRALAB+, Contract no. 654110, the U.S. Department of Energy through grant ORNL-4000171342, and the U.S. National Science Foundation through CAREER Award IIS-1943199 and grant CCF-1813624.