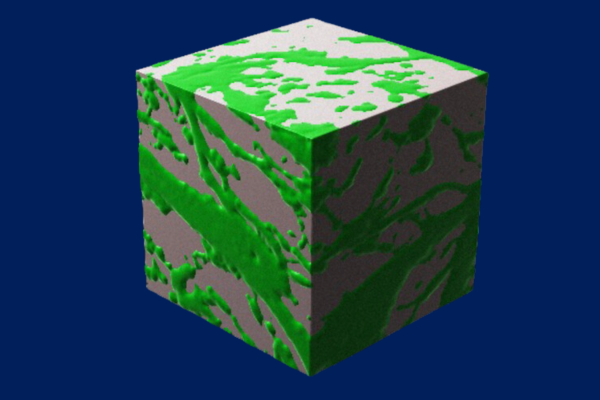
One of the most important but least understood aspects of healing is cell migration, or the process of cells moving from one part of the body to another. “If you are an ambulance out in the woods,” says Karen Xu, an M.D/Ph.D. student in Medicine and Bioengineering, “and there are no paths for you to move forward, it will be a lot harder for you to get to a site that needs you.”
Earlier this year, Xu co-authored a paper in Nature Communications describing a new cue to help cells get to where they need to go: a material made chiefly of hyaluronic acid and gelatin, two gooey substances commonly found outside cells in joints and connective tissue.
“Hundreds of thousands of people tear their meniscus every year,” says Robert Mauck, Mary Black Ralston Professor in Orthopaedic Surgery in Penn Medicine and Professor in Bioengineering at Penn Engineering and one of Xu’s advisors, as well as a senior author on the paper. “This material could potentially speed up their recovery.”
What makes the material — known as a hydrogel due to its blend of gelatinous matter and water — unique is that the combination of hyaluronic acid and gelatin forms a complex network of paths, providing cells many different ways to travel between two points.
This property is known as bicontinuity, and is exemplified by two discrete continuous phases that are each connected throughout the entire volume of the material (for example with a sponge, with phases of cellulose and air; in the hydrogel, this is comprised of gelatin and hyaluronic acid) resulting in a dizzying array of patterns that dramatically increase the surface area inside the material.

To test the hydrogel’s efficacy, Xu and her collaborators — including co-advisor Jason Burdick, formerly the Robert D. Bent Professor in Bioengineering at Penn Engineering and now the Bowman Endowed Professor at the University of Colorado Boulder, and the paper’s other senior author — first created several different versions of the hydrogel to find the sweet spot at which the constituents formed the bicontinuous structure and had the highest internal surface area. “We found that a precise combination of the various hydrogel components and control over their mixing was needed to form the bicontinuous structure,” says Burdick.
Then, the researchers embedded clusters of cells commonly found in connective tissue in the hydrogel, and watched to see how the cells dispersed. In the hydrogels with the highest internal surface area, cells moved roughly three times faster than in pure gelatin, showcasing the material’s ability to speed cell migration.
The researchers also placed samples of meniscus tissue on top of the hydrogel and tested the hydrogel in animal models. In each case, the new hydrogel measurably accelerated cell movement. “The bicontinuous structures have this extensive surface area,” says Xu, “and cells find the interfaces between the different components of the hydrogel and migrate more quickly.”

In the future, the team hopes to test the hydrogel’s clinical applications. “This could work for musculoskeletal tissues,” says Xu, such as damaged knee ligaments, but could support any tissue that needs to be repaired by nearby cells. “If cells are not able to actually get to where an injury occurred and populate that space, then the body can’t repair itself,” she adds.
In theory, the hydrogel could also be used to investigate diseases. “You can use it as a platform to better understand how cancer cells move,” says Xu. Breaking down the movement of cancerous cells could lead to treatments that hinder their movement, slowing metastasis.
Ultimately, the hydrogel promises to unlock new possibilities for engineers and physicians to direct cells, much like dispatchers phoning first responders: “Even more routes means that the ambulance can theoretically get to its final destination faster,” says Xu.
This study was conducted at the University of Pennsylvania School of Engineering and Applied Science, the University of Pennsylvania’s Center for Engineering Mechanobiology and Penn Medicine and supported by grants from the National Institutes of Health (R01AR056624, R01AR077362, R01CA221346, F30AG074508, P30 AR069619) and the National Science Foundation (CMMI-1548571, CMMI-1751898).
Additional co-authors include Nikolas Di Caprio, Mohammad Dehghany, Vivek Shenoy and Yuqi Zhang at Penn Engineering; Lorielle Laforest at Penn Medicine; Hooman Fallahi and Lin Han, Drexel University; Matthew Davidson at the University of Colorado Boulder; and Brian C.H. Cheung and Mingming Wu at Cornell University.