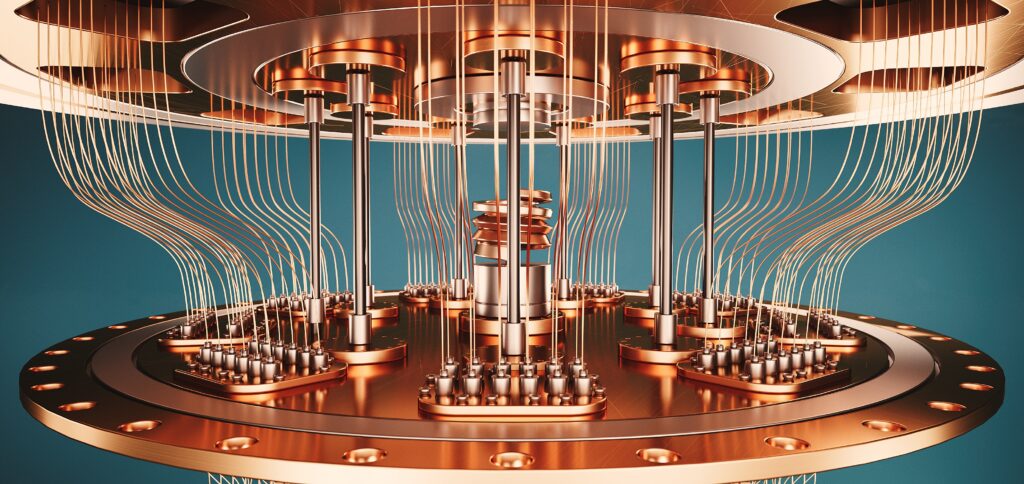
In 2018, a discovery in materials science sent shock waves throughout the community. A team showed that stacking two layers of graphene—a honeycomb-like layer of carbon extracted from graphite—at a precise “magic angle” turned it into a superconductor, says Ritesh Agarwal of the University of Pennsylvania. This sparked the field of “twistronics,” revealing that twisting layered materials could unlock extraordinary material properties.
Building on this concept, Agarwal, Penn theoretical physicist Eugene Mele, and collaborators have taken twistronics into new territory. In a study published in Nature, they investigated spirally stacked tungsten disulfide (WS₂) crystals and discovered that, by twisting these layers, light could be used to manipulate electrons. The result is analogous to the Coriolis force, which curves the paths of objects in a rotating frame, like how wind and ocean currents behave on Earth.
“What we discovered is that by simply twisting the material, we could control how electrons move,” says Agarwal, Srinivasa Ramanujan Distinguished Scholar in the School of Engineering and Applied Science. This phenomenon was particularly evident when the team shined circularly polarized light on WS₂ spirals, causing electrons to deflect in different directions based on the material’s internal twist.
The origins of the team’s latest findings trace back to the early days of the COVID-19 pandemic lockdowns when the lab was shut down and first author Zhurun (Judy) Ji was wrapping up her Ph.D.
Unable to conduct physical experiments in the space, she shifted her focus to more theoretical work and collaborated with Mele, the Christopher H. Browne Distinguished Professor of Physics in the School of Arts & Sciences. Together, they developed a theoretical model for electron behavior in twisted environments, based on the speculation that a continuously twisted lattice would create a strange, complex landscape where electrons could exhibit new quantum behaviors.
“The structure of these materials is reminiscent of DNA or a spiral staircase. This means that the usual rules of periodicity in a crystal—where atoms sit in neat, repeating patterns—no longer apply,” Ji says.
This story was written by Nathi Magubane. To read the full article, please visit Penn Today.